What is a gravitational-wave background?
Gravitational waves are fluctuations of spacetime predicted by Einstein’s general theory of relativity, which describes how gravity works. These waves are expected to be produced by almost everything in the Universe, including us human beings. Most of these gravitational waves are far too weak to be observed in laboratory experiments. However, theoretical calculations predict that we will likely be able to detect gravitational waves created by the motion of very massive stars. In general, the gravitational-wave signals from massive stars are expected to have a well defined form such as a chirp or sinusoid. If the number of such sources is very large, the gravitational-wave signals from all those sources would overlap producing a random gravitational-wave background. This is similar to hearing conversations in a crowded room. While we can clearly hear the words of loud people, we would also hear a noisy background due to the mixing of words from all other people. Apart from the gravitational-wave background from massive stars, we also might detect a gravitational-wave background from the early moments of the Big Bang when the Universe was very chaotic. In this case there are no individually identifiable loud gravitational-wave signals but only a characteristic ‘hiss’ produced by all the random processes in the Universe.
What can they tell us?
Depending on when they were produced, gravitational-wave backgrounds can be classified into two categories: cosmological and astrophysical. Cosmological gravitational-wave backgrounds are produced by sources that existed in the early Universe just a few seconds after the Big Bang while astrophysical gravitational-wave backgrounds are produced by systems of massive stars such as neutron stars and black holes that we see today. The strength of the gravitational-wave background at different frequencies strongly depends on the type of sources that produce them. Thus, depending on the type of gravitational-wave background we detect, we may learn about the state of the Universe just a few moments after the Big Bang or how the Universe is evolving in more recent times.
How do we detect them?
Since gravitational-wave backgrounds are random in nature, it is hard to search for them using data from a single detector whose noise (both inherent as well as due to the local environment) is also expected to be random. Hence searches for gravitational-wave backgrounds are done by comparing (“correlating”) data from pairs of detectors. A random gravitational-wave signal would appear the same (“correlated”) in both the detectors, and we can use this similarity (“correlation”) to distinguish it from noise from the local environment. However, the similarity is reduced as the distance between the two detectors increases. Thus, a “co-located” detector pair (two detectors in the same location) has better sensitivity to gravitational-wave backgrounds than a widely separated detector pair. Until now, all gravitational-wave background analyses 1,2,3 with the LIGO-Virgo detectors used widely separated detectors since the noise from their local environments are not correlated. We are now reporting the first analysis using the two co-located LIGO detectors, yielding a more sensitive measurement.
Did we detect any gravitational-wave background?
For this analysis, we used the data from two LIGO detectors, called H1 and H2, that were located at the same facility at Hanford, WA. Since the two co-located detectors share a common environment, this pair was more susceptible to correlated local environmental noise that could mimic a gravitational-wave signal. Hence, we needed to develop and apply new techniques to identify and remove frequencies and times with significant correlated noise from the environment. These new techniques used the data from monitoring instruments such as seismometers (used to measure the shaking of the ground), microphones (used to measure sound waves), and magnetometers (used to measure magnetic fields). After applying the new noise mitigation techniques, the data showed no evidence of correlated environmental noise at high frequencies (460 – 1000 Hz), making this a “clean” frequency range in which to look for a gravitational-wave background signal. However, we found no evidence for such a signal in our data. In other words, the H1 and H2 detectors appeared to only have uncorrelated intrinsic noise in that frequency range.
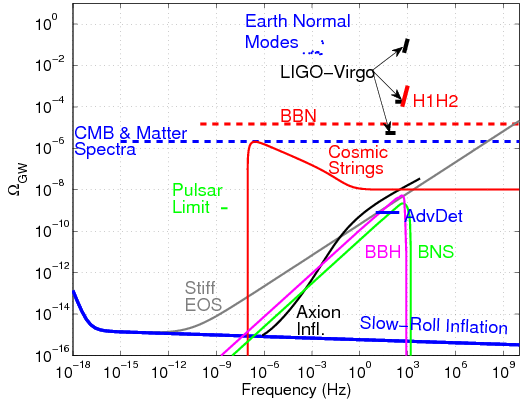
The above plot shows our upper limit (red curve labeled ‘H1H2’) on the strength of the gravitational-wave background as well as limits and predictions from various other experiments and theoretical models. The strength of the gravitational-wave background represents the fraction of the total energy density of the Universe contained in such a background. The black lines labeled as ‘LIGO-Virgo’ correspond to limits from recent LIGO-Virgo analysis using widely separated detectors. That LIGO-Virgo analysis searched for astrophysical gravitational-wave background (slanted line) as well as cosmological gravitational-wave background (horizontal line) while the current co-located ‘H1H2’ analysis focuses only on astrophysical gravitational-wave background. For the astrophysical gravitational-wave background, the upper limit from this analysis is ~180 times better than the corresponding limit from LIGO-Virgo analysis. The plot also shows the expected sensitivity of advanced LIGO and Virgo detectors (blue line labeled ‘AdvDet’).
Since we did not see a signal, we were able to put an upper limit of on the strength of the possible high-frequency gravitational-wave background that was ~180 times better than the recent LIGO-Virgo result using widely separated detectors (see Figure 1). We also performed an analysis at low frequencies (80 – 160 Hz) searching for a cosmological gravitational-wave background. However the analysis was dominated by instrumental correlations and hence we could not set any upper limit in that case. Even though we did not see any signal, we expect the new techniques developed here will be useful in the advanced detector era when even the widely separated detectors could be affected by global magnetic fields. With the expected improvement in the sensitivity of advanced LIGO and Virgo detectors as well as other global detectors, we intend to continue searching for gravitational-wave in the coming years.