Using data taken between July 2009 and October 2010, researchers from the Laser Interferometer Gravitational-wave Observatory (LIGO) Scientific Collaboration and the Virgo Collaboration have completed a joint search for merging binary star systems consisting of neutron stars and black holes.
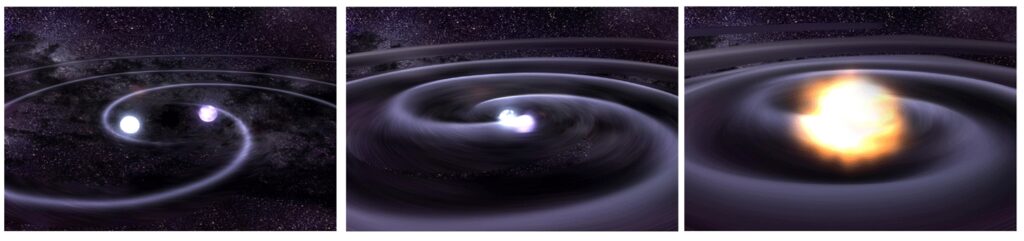
An artist’s impression of two stars orbiting each other and progressing (from left to right) to merger with resulting gravitational waves. [Image: NASA]
Neutron stars are formed when old massive stars collapse under their own gravity. As their name indicates, neutron stars consist almost entirely of neutrons packed tightly together and are extremely dense. Black holes are formed from the collapse of even more massive stars; they are so compact that even light cannot escape their gravitational pull. Black holes and neutron stars can sometimes form binary systems, that is, two neutron stars or two black holes or a neutron star and a black hole may be close enough in space to orbit around each other. As they orbit each other, the system loses energy in the form of gravitational waves. The objects move closer together and eventually merge to form a single black hole. As they “inspiral” into each other, their relative velocity increases; by the time the objects are close to merging they are moving so fast that the gravitational waves can be detected by ground-based detectors on Earth, even though the binary may be hundreds of millions of light years away. Binary neutron stars and binary black holes are one of the most promising sources for the first detection of gravitational waves.
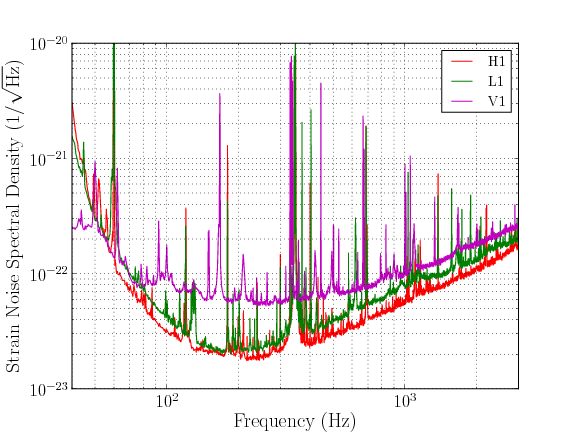
Plot showing the typical sensitivity of the three detectors used in this search. The vertical axis gives the average “strain noise” in the detectors, which is a measure of how much the mirrors typically move as a function of frequency (horizontal axis). A strain of 10-21 corresponds to a relative mirror displacement of about 10-18 meters in LIGO, or about one thousandth the diameter of a proton. A gravitational wave must create a strain in the detector larger than the strain noise to be detectable.
Scientists know that such systems exist, as astronomers have observed binary neutron stars in the Milky Way galaxy using radio telescopes. Although none of the observed binaries are close enough to merger to be detected by LIGO and Virgo, scientists can use these observations to determine the rate of mergers in the universe. Observations indicate that a neutron star-neutron star merger occurs on average only every 10,000 years in a galaxy like the Milky Way. Binary mergers do not occur very often in our own galactic backyard! By comparing the sensitivity of the LIGO and Virgo detectors to this rate, the number of possible gravitational-wave detections in a period of time can be estimated. At the same time, observations by the LIGO and Virgo detectors can set limits on the rate of mergers, which helps astronomers to make better models of the universe. A direct gravitational-wave detection would instead allow scientists to shed light on the internal structure of neutron stars and test how gravity behaves when it is very strong.
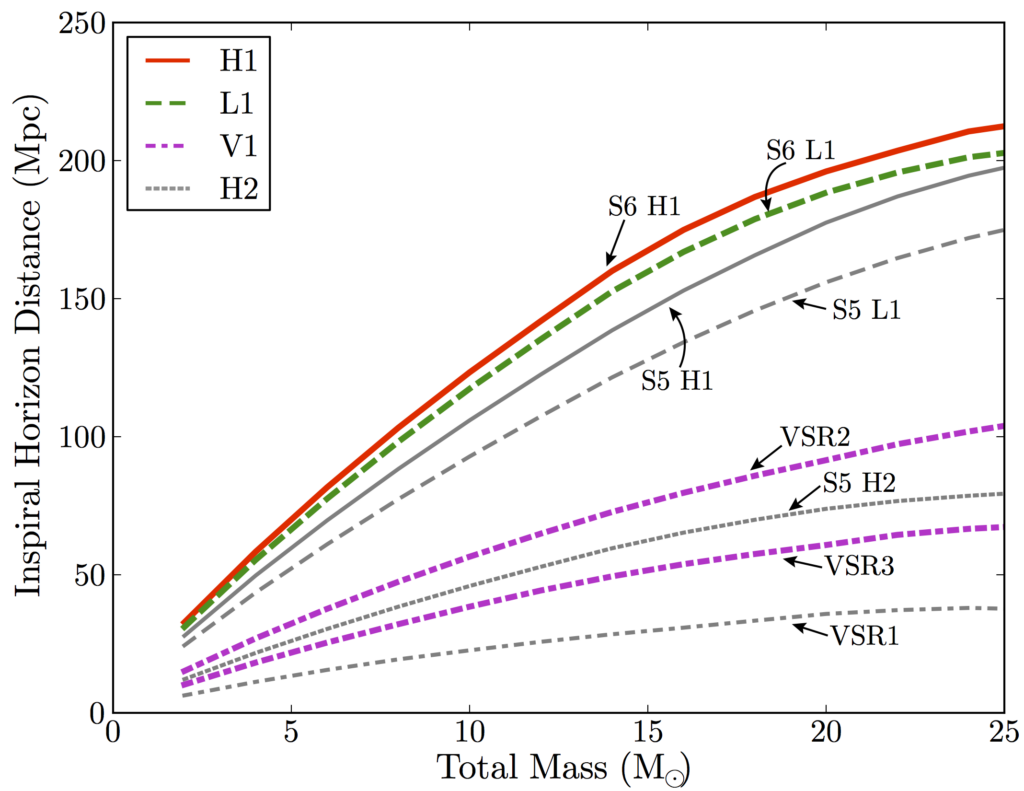
Sensitivity of each of the detectors used in this search (colored lines) compared to the most-sensitive previous search (gray lines). The vertical axis gives the distance to which a binary could be detected by each of the detectors in units of Mpc (1 Mpc is approximately 3 million light years) as a function of total mass of a binary, in units of solar masses (horizontal axis). Comparing the colored lines to the gray lines we see that the detectors in this analysis were more sensitive than in previous searches.
Prior to this search, there have been five searches for neutron star and black hole binary systems using the LIGO detectors and one search using the Virgo detector. During this new search the network of LIGO and Virgo instruments were more sensitive than ever before; they could detect binary neutron stars up to approximately 130 million light years away and binary black holes up to approximately 290 million light years. Combing through the data, LIGO and Virgo Scientists found a signal that looked like a gravitational wave from a black hole orbiting another black hole or a neutron star. It was later revealed that this signal was a “blind injection” — a fake signal secretly added to the data! The success of this exercise confirmed that LIGO and Virgo scientists are ready to detect real gravitational wave signals and tested the procedures that are used in their searches.
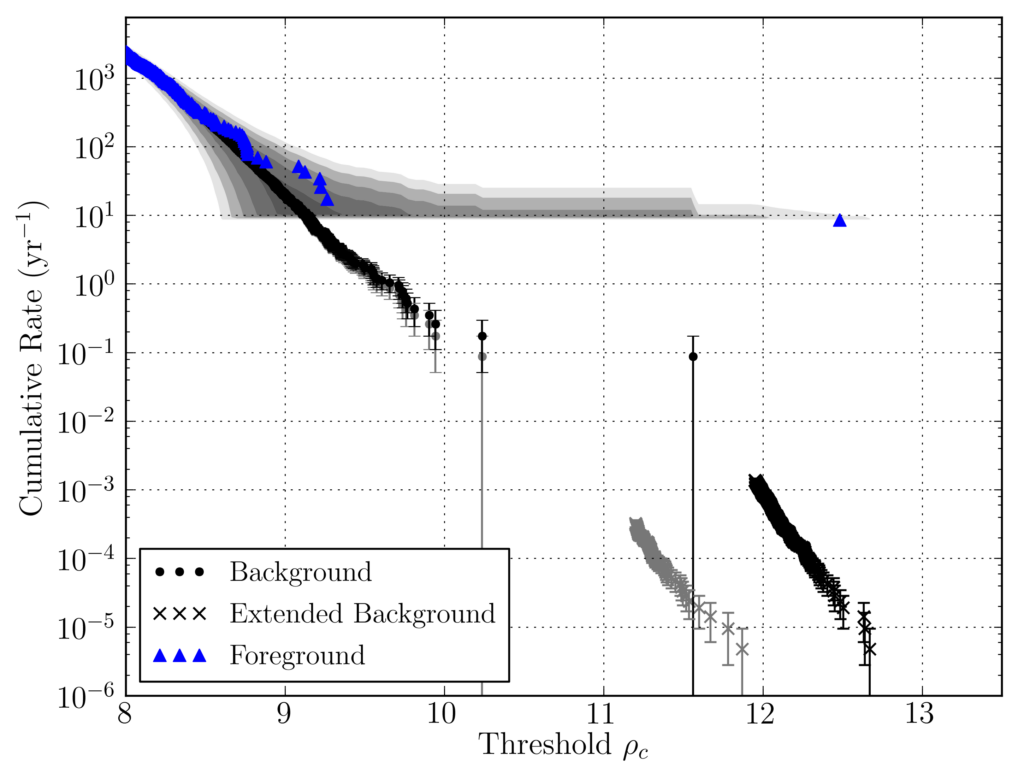
Detection plot showing the blind injection. This plot shows the rate of events per year as a function of significance. The black dots and crosses show non-gravitational wave (“noise”) events. The blue triangles show potential gravitational-wave candidates. Some of these blue triangles may be from gravitational waves, others may be from noise. The farther above the black dots a blue triangle lies, and the farther it is to the right, the more likely it was caused by a gravitational wave. The blue triangle farthest to the right is from the blind injection.
After the blind injection was removed from the data, no gravitational-wave signals were identified. This “null result” allows LIGO and Virgo scientists to set new limits on the rate of compact binary mergers in the universe. These limits are still about 100 times higher than expected rates from astronomical observations, so the fact that no gravitational waves were detected is consistent with expectations. This search is one of the last to use data from the “initial” detector era. Advanced LIGO detectors will be operational in 2015, and once these reach design sensitivity scientists will be able to detect neutron star-neutron star mergers within a volume that is 1000 times larger than initial detectors. Based on astronomical observations this means we may detect tens of gravitational waves per year. The successful identification of the blind injection as a gravitational-wave candidate in this analysis gives scientists the confidence that they will be ready — and able — to detect gravitational waves from binary neutron stars and binary black holes in the advanced detector era.
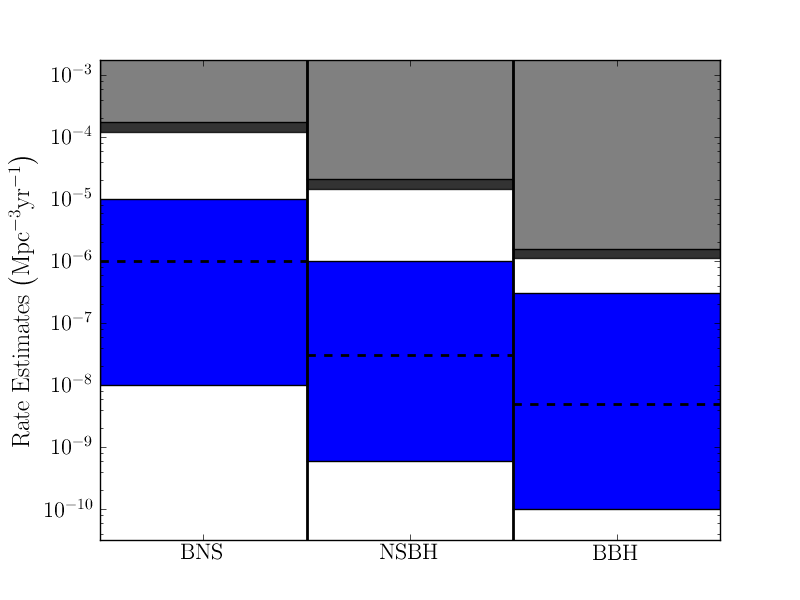
A comparison of the merger rates predicted by astronomers to the upper limits determined by LIGO and Virgo. The vertical axis gives merger rates per volume of the universe per year. The horizontal axis gives the type of system considered: a binary consisting of two neutron stars (BNS), a binary consisting of a neutron star inspiraling into a black hole (NSBH), and a binary consisting of two black holes (BBH). The light gray region gives the upper limit on these mergers determined by all prior LIGO and Virgo searches; the dark region gives the new upper limits determined by combining results from this most recent search. The blue regions show the range in astronomical estimates of these mergers, with the dashed black line showing the best estimate. Since the gray regions are above the blue regions, the fact that we did not detect any gravitational waves in this search is consistent with astronomical predictions.