Background
The discovery of GW170817 demonstrated the profound scientific implications of jointly observing gravitational waves (GWs) and electromagnetic (EM) emissions from a neutron star (NS) merger. This discovery involved a massive effort of coordination between the LIGO-Virgo-KAGRA Collaboration and the astronomical community. Binary mergers that involve a neutron star component, such as binary neutron star (BNS) mergers or neutron star-black hole (NSBH) mergers, are expected to produce detectable EM emission and have a variety of associated EM counterparts. The brightest EM component is produced by a relativistic jet material launched perpendicularly to the binary orbital plane and accelerated close to the speed of light. The relativistic jet first releases its energy in the form of a gamma-ray burst (GRB) and later shines at different wavelengths when it decelerates into the interstellar medium, the afterglow phase. The short duration of merger counterparts makes their detection challenging and requires an immediate mobilization of the astronomical community to start follow-up campaigns.
The Neil Gehrels Swift Observatory (Swift)
In the context of the detection and identification of the EM counterpart of merging compact stars, the Neil Gehrels Swift Observatory (Swift) has a cardinal role. Swift is optimized to autonomously detect and follow-up GRBs, with the help of three onboard instruments: the Burst Alert Telescope (BAT), the X-Ray Telescope (XRT) and the Ultraviolet/Optical Telescope (UVOT). The BAT is sensitive to the gamma-ray emission produced in the initial phase of the GRB, and the XRT and UVOT are responsible for the detection of the afterglow emission. In addition to being able to detect the GRB emission connected to the mergers, the Swift-BAT instrument has the unique ability to precisely determine the position of the source in the sky. The position is promptly conveyed to the astronomical community, enabling ground-based and space-based telescopes to start follow-up observations to fully characterize the multi-wavelength behavior of the transient.
Since the expected EM emission from counterparts of binary mergers can be, in general, faint, this motivates astronomers to carry out sensitive targeted searches that can recover these faint targets. As the name says, we need an astronomical GRB target, along with information about its location in space and time. The term subthreshold indicates that, thanks to a deeper statistical analysis, we are able to find sources which are nominally below the onboard Swift-BAT detection threshold. Another crucial point is that Swift has a very limited capability to store large amounts of data products onboard. Hence it is essential to promptly request data downlink for targeted analysis. This task has been successfully achieved by the construction of the GUANO pipeline, which autonomously receives trigger alerts from events of astrophysical interest (such as GRBs, fast radio bursts, high-energy neutrinos, and including GWs) and immediately requests the downlink of the relative data.
The relevant impact of any targeted subthreshold analysis is that, even if the external trigger is not significant enough to be classified as a detection, a more sensitive offline search is capable of potentially elevating the significance. In this way, such an analysis gives the possibility to find coincidences between external triggers and gamma-ray signals detected by Swift-BAT, which otherwise would have been lost. This principle has been extensively and systematically applied to all the GW triggers that GUANO was able to receive during the third LVK observing run, totalling around 1500 alerts. The ultimate goal of the analysis performed as part of this paper is to search for any possible emission in the Swift-BAT instrument which could have a spatial and temporal overlap with the GW event. The overlap is required to ensure that both the GW and gamma-ray signals are generated in the same location of the sky and within a time delay that is compatible with our theoretical expectations.
The subthreshold analysis of Swift-BAT data is carried out using NITRATES, a novel framework that has been demonstrated to possess a much superior sensitivity in comparison to the conventional BAT imaging technique. NITRATES enables the recovery of weak gamma-ray signals even if they occur outside the BAT instrument coded field of view. Running the NITRATES analysis is computationally challenging because of the complex association between the BAT response and the incoming direction of the photons. An end-to-end NITRATES run for a single trigger requires up to 500 core hours (if a standard laptop has only 8 cores, this would take almost 3 days of uninterrupted computation). Since GUANO currently ingests an average of 20 triggers per day, the use of high-performance computing clusters is essential.
Results
To claim a detection of a weak signal over background noise, it is crucial to characterize the noise properties to the best possible extent. Any artifact that can mimic an astrophysical event must be identified and rejected.
Unfortunately, the search brought no significant associations between the analyzed GW candidates and the signals collected by Swift-BAT. This is not surprising, since the great majority of the GW events analyzed with NITRATES are binary black hole (BBH) mergers. Indeed, according to the state of the art theoretical models, only in exotic scenarios do BBHs have associated EM emission. Just a handful of GW events that were followed up as part of this analysis have at least one NS component, but the associated probability of them being of astrophysical origin is less than 50%.
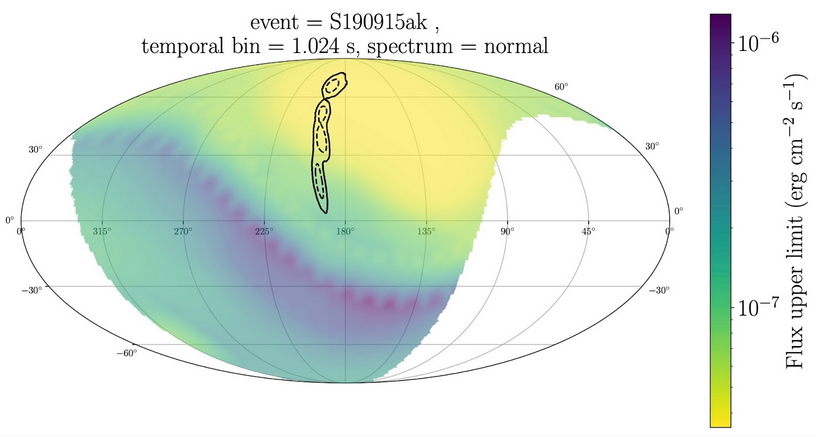
Fig.1: An example of a map of the sky accessible to Swift-BAT at the moment of the GW trigger. The color of the map indicates the flux upper limits for each sky position corresponding to a single GW event. The yellow region roughly corresponds to the most sensitive portion of the BAT instrument, providing the best possible flux constraints. The area inside the black contours indicates the region of the sky with the highest probability of containing the GW event. The white region represents the sky area blocked by the Earth.]
The natural question is: what can we do once we fail to obtain any possible association between the detected GW and gamma-ray signals? In absence of a detection, the usual practice is to determine a constraint on the intensity of any possible gamma-ray signal. Since the probability of gamma-ray detection increases with its intensity, a non-detection implies an upper limit on its brightness. Since we have full knowledge of the sensitivity of Swift-BAT, depending on the location in the sky of the putative gamma-ray signal, we can derive a sky map of the gamma-ray flux upper limit, as shown in Fig. 1. The map tells us that, in a time window of 20 seconds before and after the GW trigger, if any gamma-ray transient occurred, the associated flux must have been below the value reported on the map. We also note that the BAT instrument cannot provide any flux constraints for the region of sky that falls behind the Earth.
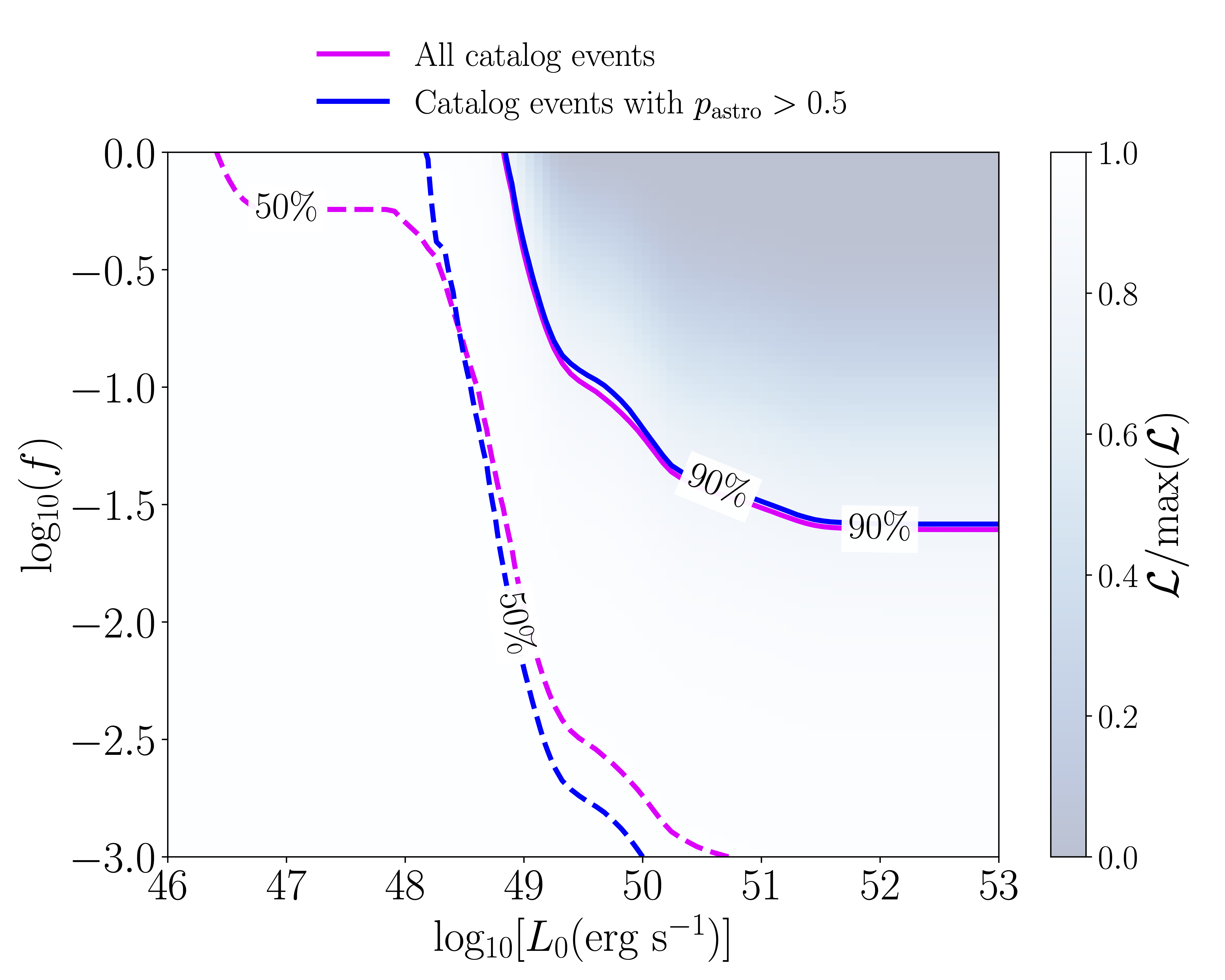
Fig.2: This figure shows the constraints on the nature of the putative EM emission from a BBH merger, using the Swift-BAT flux upper limits collected during the third LVK run. The f and L0 parameters describe the EM emission model and the regions above the lines are excluded by the analysis. Here f is the probability of having an EM emission and L0 is the typical luminosity of such an emission. The blue lines indicate the exclusion region if we consider only GW events with high probability of being astrophysical, while the pink lines are relative to an analysis that considers all the BBH events collected in O3.]
The analysis of these individual GW events provides another crucial piece of information: the source distance. With a distance and a flux upper limit in our hands, we can directly probe the intrinsic brightness of the EM emission produced by the merger of compact stars. Similar to the previous case, a non-detection leads us to conclude that the gamma-ray flash, if produced by a compact star merger, cannot exceed a maximum luminosity. Specifically, we focused on the possible EM emission from the BBH class, since they are, among all the collected GW triggers, the ones with largest astrophysical probability.
While highly improbable, several theoretical models predict EM emissions from BBH mergers in the gamma-ray band. For example, if the BBH merger happens in a dense environment, like the disk of an active galactic nucleus, theoretical models predict a possible detectable EM transient. In this work, we consider a model with minimal assumptions on the nature of the EM emission. The model characterizes the EM emission only with two quantities: f and L0, where f is the probability of having an EM emission and L0 is the typical luminosity of such an emission. Performing a simulation that incorporates all our knowledge on the Swift-BAT flux upper limits, the distance and the plausibility of the astrophysical origin of the GW event itself, we can infer constraints on the two parameters f and L0, as shown in Fig. 2. As evident from the figure, the two parameters are degenerate, therefore two possible scenarios could explain the results. The first possibility is that a large fraction of BBH mergers can produce an EM emission, but in this case such an emission cannot be too bright. Alternatively, a bright gamma-ray flash could potentially be produced by BBH mergers, but only very rarely (f is much smaller than one).
Future prospects
This work demonstrates that even in the absence of any joint significant detection, a sensitive targeted analysis can provide valuable insights into our theoretical expectations regarding the nature of EM counterparts of merging compact stars.
The subthreshold analysis pipeline used in this work adopts an extremely efficient workflow. Due to the availability of multiple supercomputing facilities, it is capable of ingesting many triggers per day. The code is also publicly available for other astronomy groups to adopt and customize as needed. It is currently ingesting several tens of GW triggers per day as part of the ongoing fourth LVK observing run. If there is a potential GW+GRB association, Swift promptly communicates with the LVK, to assess its joint significance. If the significance passes a pre-established threshold, a public alert is issued, including basic information about the nature of the GW, the gamma-ray flash and respective localizations in the sky. The alert, received promptly by the astronomical community, can trigger further follow-up of the event, to detect any possible emissions across the entire EM spectrum.
Swift‘s rapid slewing and arc-minute precision localization capabilities position it as one of the leading discovery machines in the current era of multi-messenger transient astrophysics. Novel targeted search methods, similar to the one utilized in this work, will set the stage for future missions to adopt similar strategies, enhancing our ability to pursue transient science and uncover the nature of compact star mergers.
Find out more:
- Visit our websites:
- Read a free preprint of the full scientific article here or on arXiv.org.
- The published scientific paper can be found here.
Back to the overview of science summaries.