Scientists expect that neutron stars and black holes — the superdense remnants of dead stars — bound together in close orbits will not stay that way forever. Over time, they will lose energy by the emission of gravitational waves and “inspiral” towards each other until they merge together. This process can generate gravitational wave signals strong enough to be detectable on Earth by the LIGO and Virgo gravitational-wave observatories. However, since the strength of the gravitational-wave signal decreases with the distance to the source, these observatories can only detect these events out to some maximal distance. A crucial input to understanding the performance of the gravitational-wave detectors is determining this maximal distance: the farther the detectors can see, the more signals we expect to detect! Furthermore, providing this information lets other scientists evaluate their models for compact binary formation to predict detection rates for a wide range of masses.
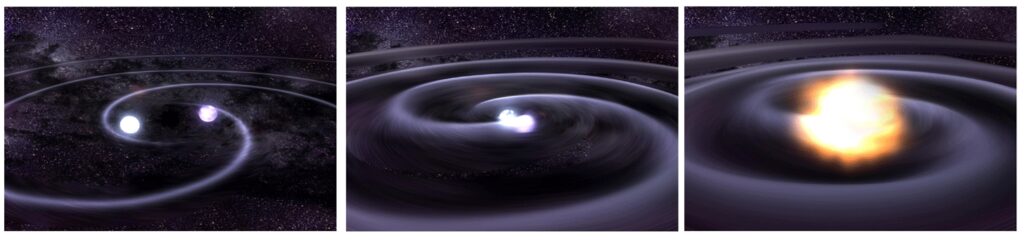
An artist’s impression of two stars orbiting each other and progressing (from left to right) to merger with resulting gravitational waves. For more information, see this introduction to gravitational waves from compact binary mergers. [Image: NASA]
The LIGO and Virgo gravitational-wave observatories have recently completed their final science runs before advanced detectors come online around 2015. A summary of the sensitivity achieved during these science runs is now publicly available. The observational periods consisted of LIGO’s sixth science (S6) run and Virgo’s second and third science runs (VSR2-3). During these runs, the instruments operated with record sensitivity to inspirals from black hole and neutron star binaries; they could detect binary neutron stars up to approximately 160 million light years away and binary black holes up to approximately 620 million light years. Astronomers often measure distances to galaxies in units of megaparsecs (Mpc). A megaparsec is one million parsecs, and a parsec is 3.26 light-years; so 160 million light years is about 50 Mpc.
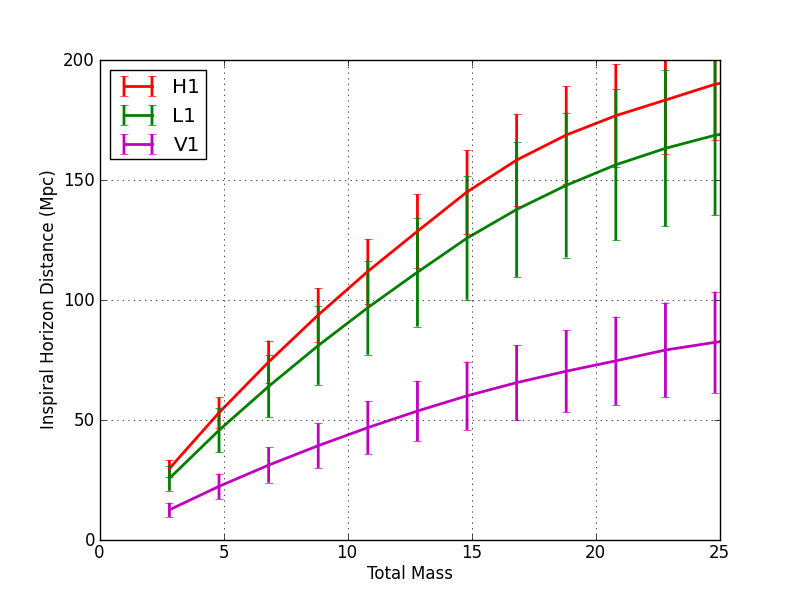
Sensitivity of each of the three detectors which ran during the S6/VSR2-3 science runs. The vertical axis gives the distance to which a binary could be detected by each of the detectors in units of Mpc (1 Mpc is approximately 3 million light years) as a function of total mass of a binary, in units of solar masses (horizontal axis).
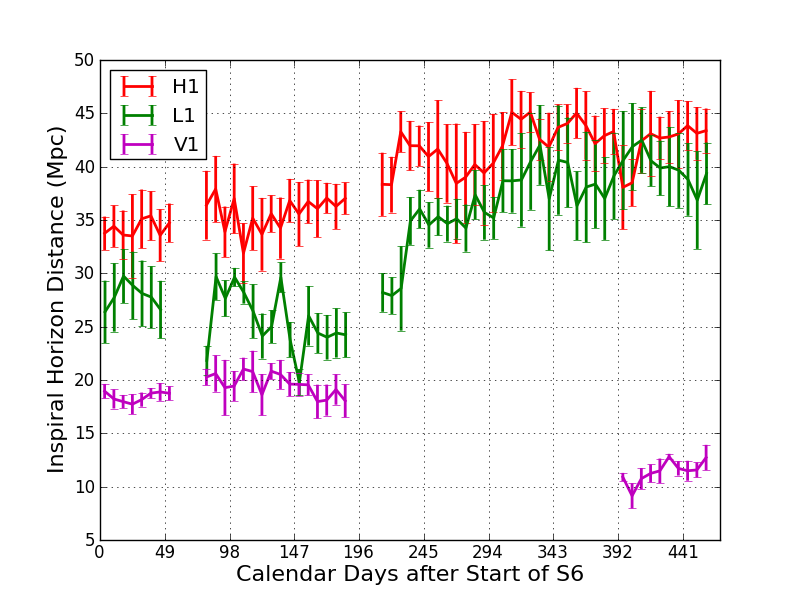
Sensitivity of each of the three detectors which ran during the S6/VSR2-3 science runs, depicting the variation in sensitivity as a function of time. The sensitivities of the detectors vary over time because of changes in local environmental conditions, such as the rise and fall of traffic. The instruments also undergo routine maintainence and upgrades throughout the run, which change the characteristics of the noise.
LIGO operated two instruments during its science run, the four kilometer detector in Hanford, Washington (H1) and the four kilometer detector in Livingston, Lousiana (L1) from July 07, 2009 to October 20, 2010. The two kilometer detector at the Hanford site, which had been included in the fifth science run, was decommissioned prior to the start of this run and did not collect data. Virgo operated its three kilometer interferometer (V1) during roughly the same timespan, except for a commissioning break between the second and third science runs, from January 11, 2010 to August 6, 2010.
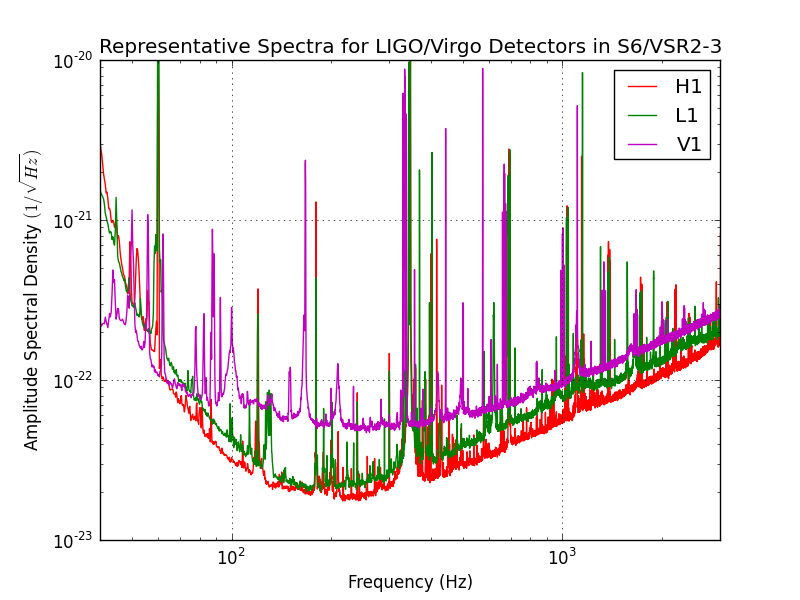
Plot showing the typical sensitivity of the three detectors used in this search. The vertical axis gives the average “strain noise” in the detectors, which is a measure of how much the mirrors typically move as a function of frequency (horizontal axis). A strain of 10-21 corresponds to a relative mirror displacement of about 10-18 meters, or about one thousandth the diameter of a proton.