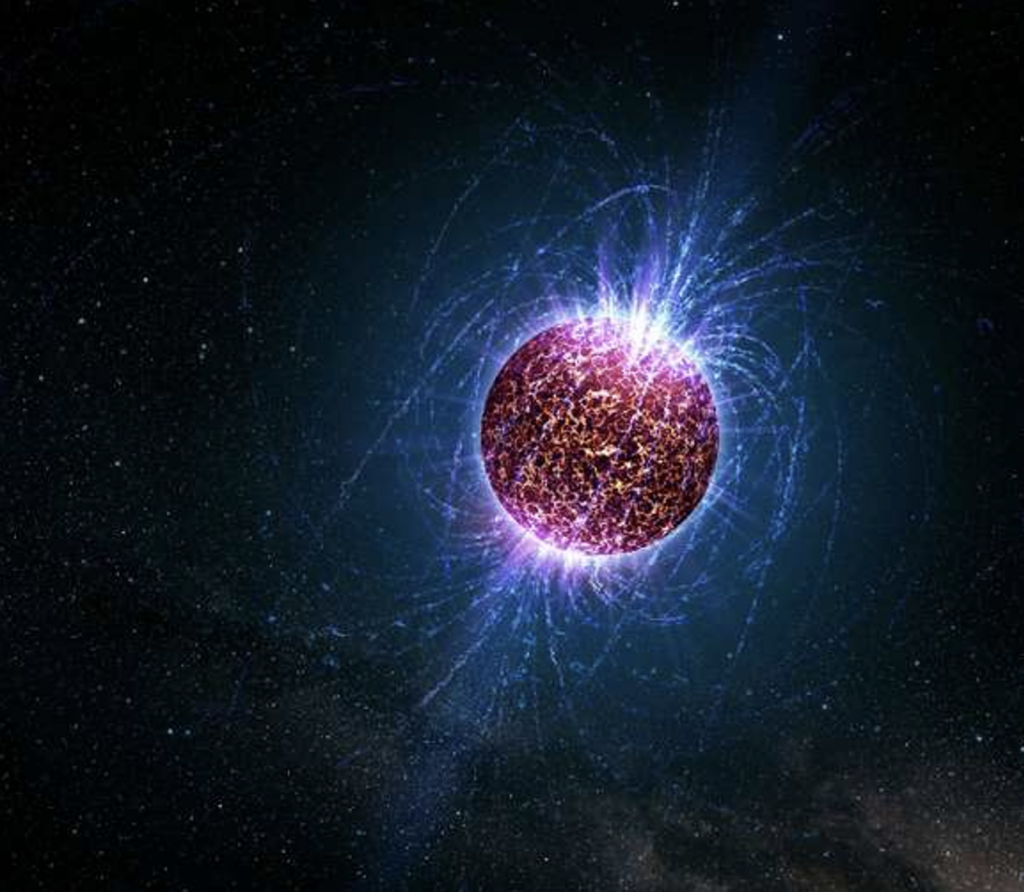
Figure 1: An artist’s impression of a magnetar, a possible source for fast radio bursts. Credit: NASA.
Fast radio bursts (FRBs) are a class of energetic radio signals, which typically last about a thousandth of a second. The vast majority of the thousands of FRBs detected arrive at Earth from very large distances outside our own Galaxy. Our ability to detect extragalactic FRBs is linked to their extremely large power: a single FRB can be about 100,000 to 10 trillion times brighter than the Sun over a fraction of a second! In a few cases, more than a single FRB flashes out from the very same place in the sky, but at different times (separated by periods of quietness lasting minutes to years), hinting that the physical processes that produce FRBs do not completely destroy their sources (in spite of the tremendous energy released).
While we do not know the precise origins of FRBs, we think that they come from powerful events in the close vicinity of neutron stars. A fraction of these neutron stars harbor the strongest magnetic fields known to exist in the Universe—about a quadrillion times (1015) that of the Earth—thus, they are conveniently called “magnetars”. The magnetic field in a magnetar threads its interior, passes through its surface, and extends to great distances from it, forming an extremely low-density atmosphere, the “magnetosphere”—similar to the Earth’s or Sun’s magnetospheres. Magnetars are physically extreme objects which not only host FRBs, but also other kinds of activity such as X-ray bursts, which are bright flashes of X-rays, and glitches, which are sudden changes of the rotation rate of the magnetar.
An important clue as to the nature of FRBs arrived when the Galactic magnetar SGR 1935+2154 produced an FRB on April 28th 2020, marking the first time an FRB came from our own Galaxy, and also the first time FRBs and X-ray bursts were observed concurrently. In fewer than three years, this special source has produced three more FRBs: on October 8th 2020, October 14th 2022, and December 1st 2022.
While FRBs and magnetar X-ray flares may be related phenomena, the same magnetar may produce FRBs without X-ray flares and vice versa. This has indeed been the case with SGR 1935+2154. A leading theory states that flares happen as a result of seismic activity in the crust of magnetars. Under sufficiently strong twisting, the crust cracks, just as the Earth’s crust does during an earthquake. The enormous energy released during the crust cracking is imparted to both the neutron star interior and its surrounding magnetosphere. The fraction of the energy that goes to the magnetosphere may ultimately produce a magnetar flare and/or trigger an FRB. The rest of the energy (actually, most of it) hits the neutron star interior, making it vibrate at very special frequencies, the so-called quasi-normal oscillatory modes.
Searching for Gravitational Waves
Gravitational waves (GWs) are vibrations in the fabric of spacetime, which can carry energy from their sources to the rest of the universe. The oscillations of the magnetar interior induced by crustal fractures are a potential source of GWs, and may be related to how FRBs are emitted. Since the properties of these GWs depend on the nature of the neutron star and its magnetic field, their observation in near-concurrency with magnetar flares is a long-sought but hitherto unrealized discovery. Some advanced theoretical models indeed predict that the energy released in GWs from internal oscillations of isolated magnetars may be relatively modest compared to detected sources of GWs (e.g., mergers of binary black holes). Thus, current GW detectors should not be sensitive enough to measure them. While a discovery of GWs in association with magnetar activity would be extremely rewarding, their non-discovery allows us to estimate an upper threshold for the energy released in GWs and, therefore, restrict the models that we employ to understand the nature of magnetars. In this paper, we report on the non-detection of GWs in association to the FRBs observed for the Galactic magnetar SGR 1935+2154.
The farther away the GW source is located, the smaller the strain. Even gigantic amounts of energy, like those released in powerful astrophysical sources, yield minuscule strains on Earth—so small that extremely sophisticated detectors have to be employed. We detect GWs using lasers that monitor the motion of suspended mirrors at the LIGO, Virgo, and KAGRA observatories, as well as the GEO600 observatory. Having more than a single GW detector observing simultaneously is a great advantage to dismiss fake GW signals. If an actual GW passes by one detector, similar imprints are expected on different detectors distributed around the Earth at slightly different times.
When an FRB occurs in a known magnetar, we search the GW data for potential GW counterparts of the observed electromagnetic signal around that time. The FRBs produced from the Galactic magnetar SGR 1935+2154 happened at times when the LIGO and Virgo observatories were offline. Fortunately, GEO600, while not as sensitive as LIGO and Virgo, was operating during three out of the four FRBs from SGR 1935+2154, and collected useful GW data.
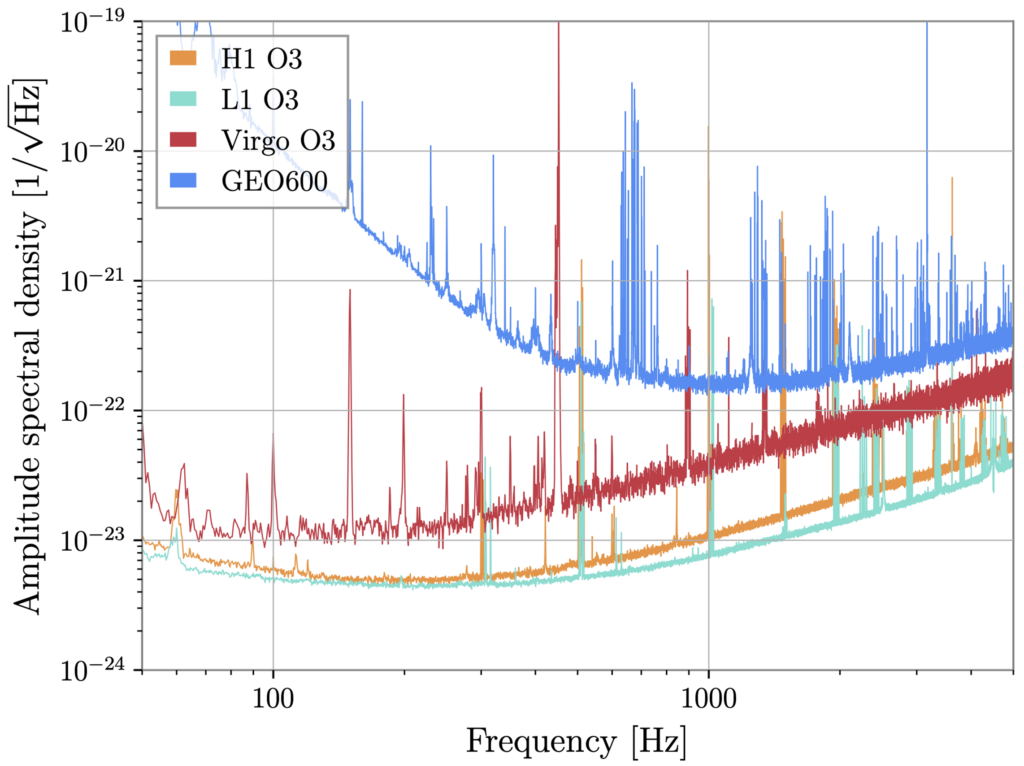
Figure 2: Sensitivity curves of LIGO (orange, teal) and Virgo (red) compared to GEO600 (blue), as a function of the GW frequency. The lower the curve, the more sensitive the detector at that frequency.
This single detector setup poses a few challenges. First, distinguishing real signals from noise becomes more complex, as explained above. Second, not being able to combine data from multiple GW observatories makes it more challenging to focus on the particular patch of sky from where the FRB came. Third, the sensitivity of GEO600 is lower than that of LIGO and Virgo detectors, which may render GWs in the frequency range below approximately 1 kHz undetectable. Fortunately, theoretical models predict that any GWs produced by quick oscillations in the magnetar’s interior would likely be at high-frequencies (1-3 kHz), where GEO600 has its best sensitivity.
Unlike the case of mergers of binary black holes, we do not know the exact form of the expected GW signals. Hence, we resort to generic “waveforms” that represent our best guess at what they may look like. We keep our search “minimally modeled,” meaning that they allow some room for detecting a signal even if that signal does not match precisely with the theoretical waveforms. We used two different software analysis packages, one for short GW signals of under one second, and another for longer signals lasting between one and ten seconds, and searched for GW signals during the FRBs and surrounding X-ray events from SGR 1935+2154.
Results and Future Prospects
Even accounting for the fact that SGR 1935+2154 is the closest source of FRBs (so far), we did not detect any GWs associated with the FRBs. In order to determine the sensitivity of our analysis, we simulated artificial data representing a GW of plausible properties, noting the amplitude (how “loud”) at which these simulated GWs become detectable by our search. We then used these amplitudes to determine upper limits on the GW emission energy: any signal would have had to be less energetic than our upper limit to remain undetectable. These limits improve on the existing upper limits, set by the LVK in an analysis of FRBs from 2019, by up to 4 orders of magnitude. We also slightly improve constraints on the ratio of energy released in GWs to that released in radio waves during the FRB.
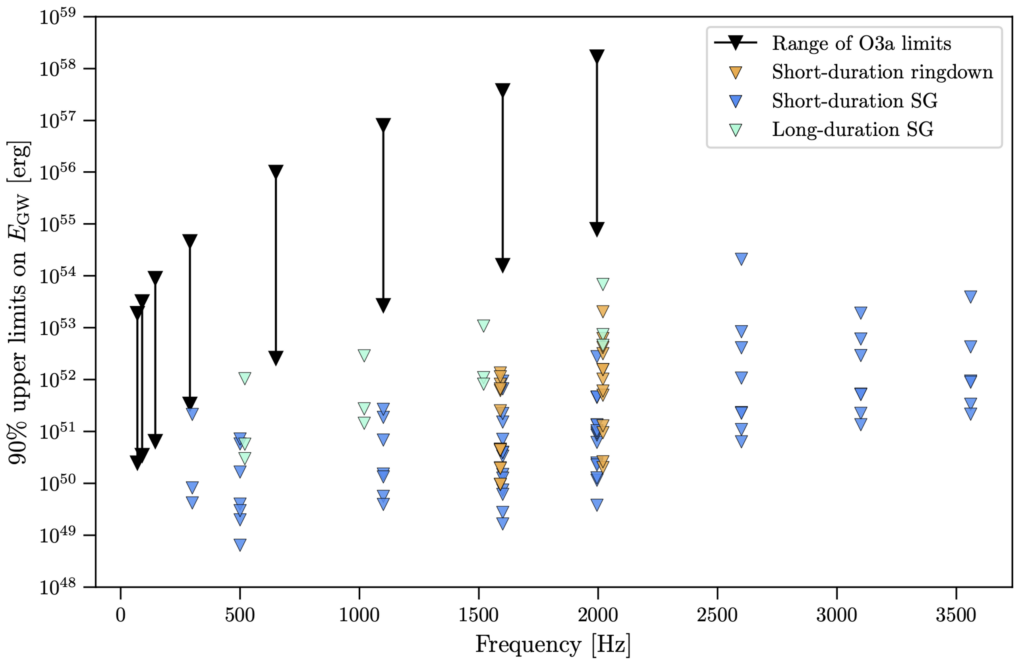
Figure 3: Upper limits on GW energy released during FRBs. Our new limits, at various frequencies and models, are plotted in colored triangles. Ranges of previous limits are in the black arrows. Our new limits are significantly more restrictive.
All existing predictions about the energy released in GWs accompanying FRBs are lower than our upper limits. Thus, our latest thresholds remain not restrictive enough to select among the various theoretical models predicting a connection between FRBs and GWs in magnetars. Regardless, these limits restrict the possible ways that GW emission can be linked with FRBs, helping theorists in their quest for improved FRB models.
Finally, since the LVK’s GW detectors will remain in observing mode until mid-2025, if SGR 1935+2154 (or even another Galactic magnetar) produces FRBs again, we may be able to search for coincident GWs with more sensitive detectors, giving us another opportunity to better understand these extreme astrophysical phenomena.
Find out more:
Back to the overview of science summaries.