Einstein’s theory of general relativity predicts that two closely-orbiting massive objects will lose energy and fall towards each other, emitting a distinct gravitational-wave signature. Black holes, the ultra-compact remnants of very massive stars, are prime candidates for emitting detectable gravitational waves in this way. While current detection work focuses on commonly-expected binary systems of black holes with masses a few times that of the Sun, it is possible that there are detectable populations of black holes which have masses larger than this—hundreds or thousands times the mass of the sun. These are dubbed intermediate mass black holes, as their masses lie in the currently-unobserved range between solar mass black holes and the supermassive black holes in the center of galaxies. The gravitational-wave interferometer network of LIGO and Virgo could be able to measure ripples from the final moments of such intermediate mass binary black holes merging together.
Intermediate mass black holes pose a mystery to astronomers, as it is difficult to explain how they could grow larger than a few times the mass of the Sun. One possibility is that intermediate mass black holes form from the deaths of huge stars present in the earliest galaxies. These stars would be hundreds of times more massive than the Sun, and their subsequent demise could leave remnant black holes with very large masses. However, to emit gravitational waves, these black holes must find a partner, which is likely a rare occurrence. Another scenario involves dense clusters of stars. It is possible that a binary intermediate mass black hole system could be built by successive mergers of many stars over time, forming one or more large black holes near the center of the cluster. This scenario provides a stronger detection possibility for gravitational-wave detectors.
Candidate intermediate mass black hole systems have been observed, but they are still relatively rare. A gravitational-wave detection of an intermediate black hole binary merger would confirm their existence and provide information on the abundance of such systems in the Universe, as well as precisely measuring the component masses. The presence or absence of these signals will shed light on star formation in the early universe, provide important information about the dynamics and structures of globular clusters, and give clues about the formation of supermassive black holes.
A search for merging intermediate mass black holes was conducted using data from gravitational-wave detectors in the joint data taking run S5/VSR1. These data were taken at two sites of the Laser Interferometer Gravitational-wave Observatory (LIGO) between November 2005 and October 2007. In May 2007, the detector operated by the Virgo Collaboration joined the search. Traditionally, searches for gravitational waves from binary systems used a technique which searches the data for the expected pattern of our signal. It is a powerful technique if one knows in advance what pattern to expect. However, other methods are also possible. This search uses a method which does not necessarily have a preference for any specific pattern. This method, usually called an “un-modeled search”, compares data from different detectors against each other and looks for any patterns consistent between all of the detectors. This is the first search ever performed for gravitational waves from sources in the intermediate mass range and is meant to complement other searches for lower mass binary compact objects.
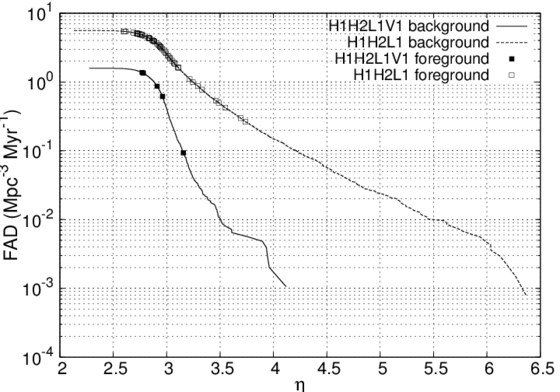
Plot used to derive the significance of the most promising events in the search. This plot compares those events to chance of obtaining events which originate from noise (background). The two curves (bottom is the four detector Hanford-Livingston-Virgo or H1H2L1V1 network, top is the three detector LIGO only H1H2L1 network) represent the relative frequency of noise “false alarms”, e.g. electronics in the detector acting up. The event candidates for either detector network (foreground) are the superimposed squares and triangles. This plot is ultimately used to understand how frequently a coincidence would appear to be a detection of the binary systems considered here, and thus allows us to quantify the significance of the events we might think are real. The points further down on the curves are events which are more likely to be real. Unfortunately, we still cannot conclude with any confidence that any of the events we considered were actual detections.
The search for intermediate mass black holes described above found no evidence for such merger events in our data. Instead, we placed experimental limits on how often they could occur given our own observations— that is, we calculated how rare the signals must be given our current ability to detect them. We found that, in a sphere of space over a billion light years across, there should be less than one event per year!
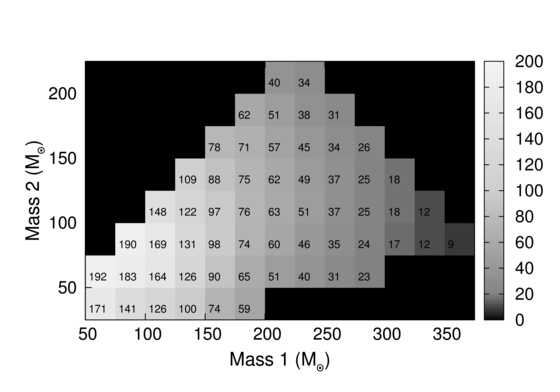
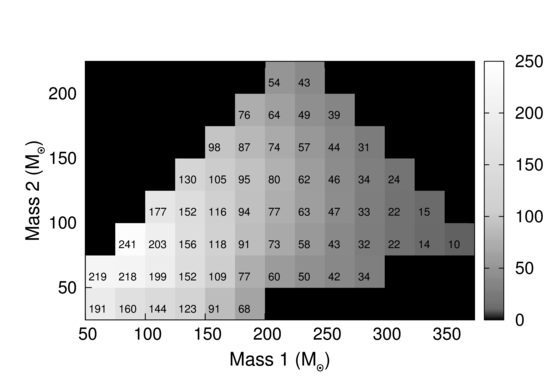
Each of the boxes in this plot indicates a region of the binary masses we looked for. The horizontal axis is the mass of one of the black holes in the pair, and the vertical indicates the other black hole mass. Within this box, the number indicates the approximate limit in how far away (in megaparsecs or about three million light years) we can detect systems with those component masses. Lighter colored boxes indicate a more distant limit. We are many times more sensitive to relatively lighter binary systems (less than two hundred times the mass of the Sun) and therefore we can “see” via gravitational waves a volume of space including a multitude of galaxies. In contrast, as the binary gets more massive or the component black holes making up the binary become less similar, our ability to detect them decreases drastically. The left plot is for the time when only the LIGO network was operating, and the right plot is for joint operation between LIGO and Virgo.
Even without a detection, is an impressive achievement to be able to peer that far into the depths of our Universe. This search only covered the first joint run between LIGO and Virgo, but a similar analysis of a second joint run that took place between 2009 and 2010 is in the works. Plans for even more advanced detectors, upgraded versions of those used in this search, should come to fruition around 2015. A network composed of these advanced detectors will be able to probe even larger mass systems and allow us to look even deeper into the Universe.