According to Einstein’s theory of General Relativity, gravitational waves are ripples in the fabric of space – a stretching and squeezing of space itself. They are caused by some of the most violent phenomena in the universe, such as the supernova explosions that blast apart dying stars, and the collisions of neutron stars and black holes. Although they were first predicted by Einstein almost a century ago, gravitational waves have not yet been directly observed. In the past decade, a dedicated network of observatories has been constructed to detect them. This network includes the Laser Interferometer Gravitational-wave Observatory (LIGO) in the United States, the British-German GEO600 detector in Germany, and the French-Italian Virgo detector in Italy. All three experiments share their data in the quest to detect gravitational waves.
Collisions of two neutron stars or a neutron star with a black hole are astrophysical phenomena which are likely to generate gravitational waves that are strong enough to be detected on Earth. (See the results of a recent LIGO and Virgo search for gravitational waves from compact binary systems.) These cataclysmic events are also thought to be the cause of some brief flashes of very energetic gamma radiation that are observed from all parts of the sky. Astronomers call these events “short gamma-ray bursts” (short GRBs), and the duration of the flash is usually shorter than two seconds. Longer-lasting gamma-ray bursts, or “long GRBs”, are thought to be produced during the explosive death throes of super-massive stars, or supernovae, and may release burst-like gravitational waves.
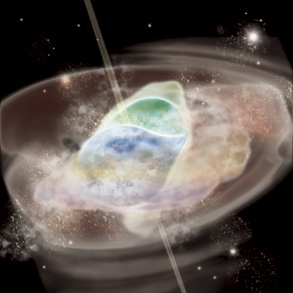
An artist’s impression of a gamma-ray burst. [Image: NASA/Swift/Aurore Simonnet]
GRBs can only be observed from space, as Earth’s atmosphere blocks gamma rays from reaching the ground. Therefore, several satellites orbiting the Earth are used to observe GRBs. The new LIGO-Virgo analysis which is reported here used data from NASA’s Swift and Fermi satellites, as well as from AGILE, INTEGRAL and the Third Interplanetary Network. The analysis covers 154 GRBs that were detected during the latest joint data-taking period of the LIGO-Virgo observatories, between July 2009 and October 2010. LIGO and Virgo scientists searched for gravitational-wave signals occurring at the same time as the observed GRBs, and from the same directions on the sky. Two searches have been performed. The first one searched specifically for a signal from the coalescence of two neutron stars, or a neutron star and a black hole, from short GRBs. The second search looked for a burst-like gravitational wave signal from the collapse of a massive star from all GRBs in the data sample. No gravitational-wave signals were found by either search around the times of the GRBs, and the LIGO-Virgo analysis concludes that on average the GRB events must have taken place at distances larger than 50-100 million light years.
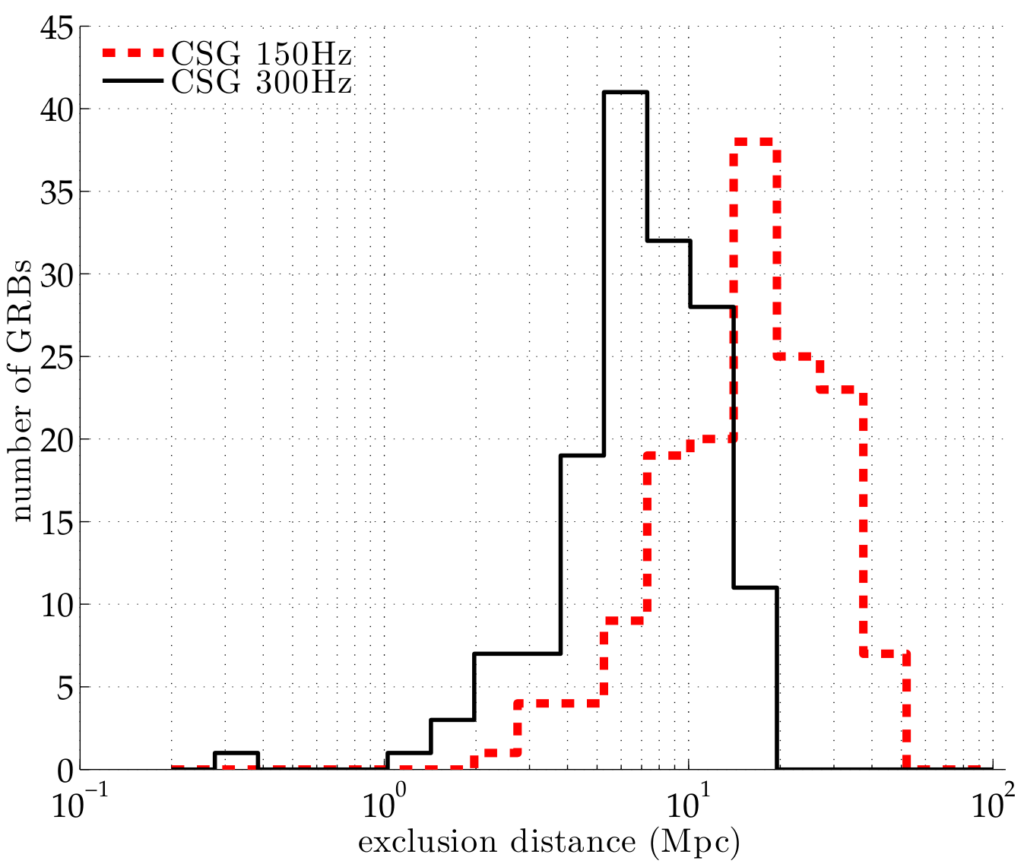
Plot showing the distribution of exclusion distances for burst signals as calculated for each of the GRBs. As we have not seen any gravitational wave signals in the data, the true source must be farther away than the exclusion distances. The exclusion distances for two different frequencies are shown, 150 Hz (red, dashed line) and 300 Hz (black, solid line). As the detectors have their maximum sensitivity around 150 Hz, one gets larger excluded distances for the 150 Hz models; the median exclusion distance is 17 Mpc (about 55 million light years) for this case. In both cases, we assume that the gravitational waves carry an energy equivalent to 1% of the sun’s mass.
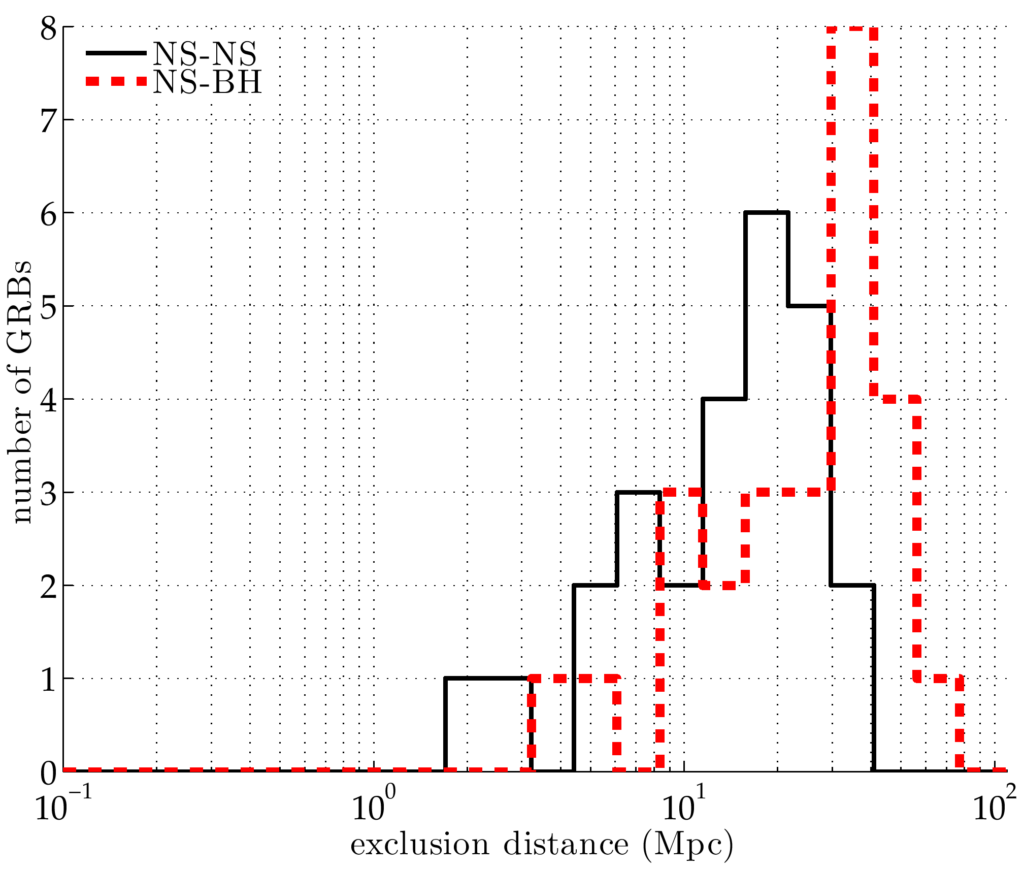
Plot showing the distribution of exclusion distances for sources of binary merger signals, either from the merger of two neutron stars (NS-NS, black solid line) or the merger of a neutron star and a black hole (NS-BH, red dashed line). As those signals can be modeled precisely, the median distance excluded for a NS-NS system is 16 Mpc (about 50 million light years) and that for a NS-BH system is 28 Mpc (about 90 million light years).
To those hoping for the first detection of gravitational waves, this “null” result may sound disappointing. As we write, however, the LIGO and Virgo gravitational-wave detectors are being upgraded to greatly improve their sensitivity. When these new advanced detectors become operational around 2015, they will be able to see 10 times further into space. Detection of gravitational waves in coincidence with GRBs may then become a reality, opening a new era of astrophysical research.
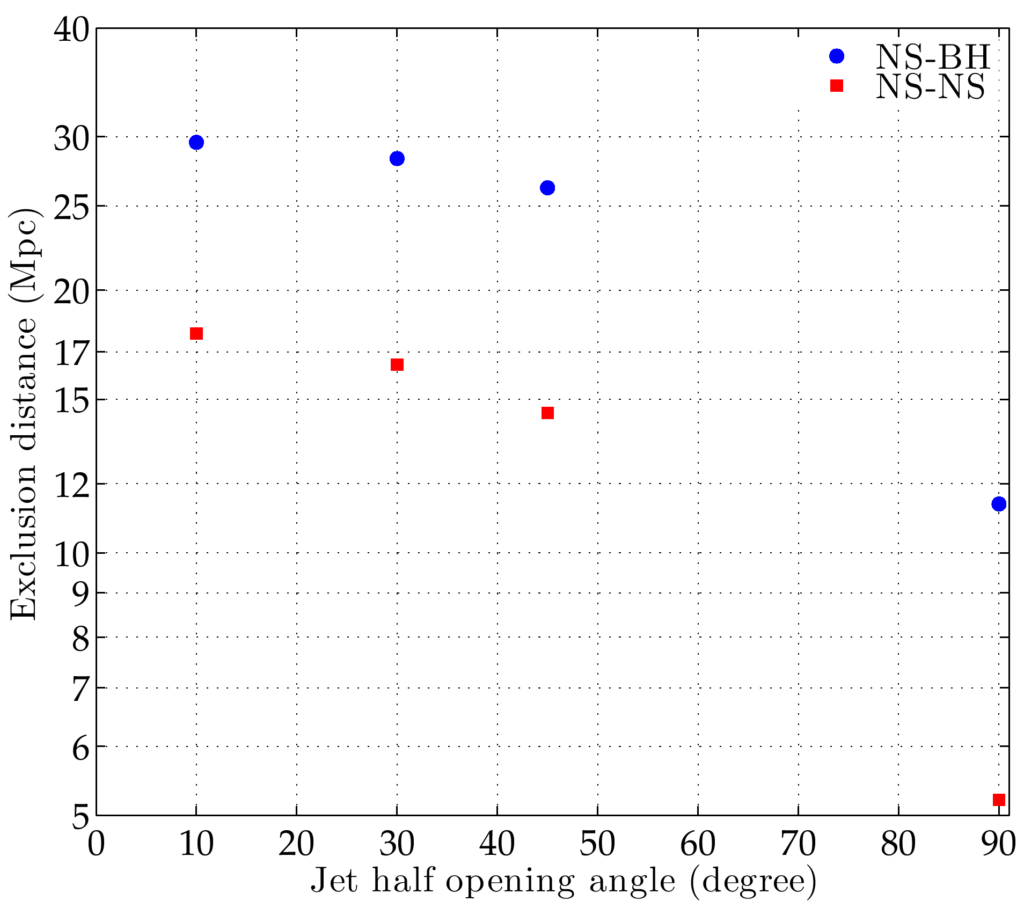
Gamma-ray bursts are believed to emit the gamma radiation only inside a cone of defined–but currently unknown–opening angle. Since we have detected the GRB, we must be inside its cone. Furthermore, the cone is most likely oriented around the rotational axis of the GRB engine, which is also the direction that is assumed to emit the maximum amount of gravitational waves. Therefore, if the opening angle of the cone is very small, we would likely receive a stronger GW signal than if the opening angle would be very large. This leads to larger exclusion distances for GRBs when no signal is observed. This relationship is depicted in this plot, which shows the size of the opening angle on the x-axis and the excluded distance on the y-axis for the two populations we are examining.
Read more:
- Freely readable preprint of the paper describing the analysis and results: “Search for Gravitational Waves associated with Gamma-Ray Bursts during LIGO Science Run 6 and Virgo Science Runs 2 and 3” by J. Abadie et al. (the LIGO Scientific Collaboration and the Virgo Collaboration).
- Web page with data used to make the plots and tables in the paper
- An introduction to gravitational waves from inspiraling binaries
- An introduction to gravitational-wave bursts