On 17 August 2017, Advanced LIGO and Advanced Virgo detected a gravitational-wave signal, called GW170817, matching the insprial of two neutron stars. Subsequent observations by astronomers from around the world revealed an electromagnetic counterpart, allowing us to pinpoint the source of the signal. The home of our binary of neutron stars is the galaxy NGC 4993. Using our observations of the source, we can try to figure out how the neutron stars formed; it seems they are probably similar to those we know in our own Galaxy.
We know that GW170817 ended with a bang. Our two neutrons stars inspiralled together, eventually merging, resulting in some celestial fireworks. But how was the binary formed?
Neutron stars are the remains of exhausted stars. When stars have burnt through their supply of fuel, they no longer have the energy to keep themselves puffy, and they start to collapse under their own weight. The central core of the star gets squeezed down, releasing energy which can blast away the outer layers of the star. Neutron stars are formed when the core is too massive to end up as a white dwarf (as our Sun will), but not big enough to end up as a black hole. Large amounts of energy are released as a star’s core forms a neutron star, resulting in a bright explosion called a supernova. Our neutron star binary started with two bangs, one for the birth of each of the neutron stars.
During a supernova, material might not explode outwards in all directions equally. This results in the newly formed neutron star getting a kick. It can be propelled by the blast at speeds of several hundred kilometres per second (equivalent to millions of miles per hour). We worked backwards from the collected observation of GW170817 to try to figure out how big a kick it could have received.
This calculation is kind of like trying to figure out how hard a pool ball was hit, given that we know it ends up in the corner pocket. It could have been cued softly, if it started close to the pocket, or hit really hard and bounced off several cushions. We can try a number of shots, from different starting positions, and see what works out. For GW170817, we simulated a large number of binaries, starting from different points in the galaxy, and given different kicks, and saw which ones ended up merging at a distance from the center of the galaxy that matches the observed position of the counterpart. The binary could have started close by and got a really small kick, but there are fewer stars formed in the outer parts of the galaxy than further away in the center; it could have formed further away and got a big kick, but if the kick is too big the two neutron stars will fly apart and never merge; it could have got a small kick and travelled a long way slowly, but then the neutron stars must not start too close to merging or they would do so before arriving at their final position. Folding together all the possibilities, we can work about the probabilities for having different kicks and starting configurations.
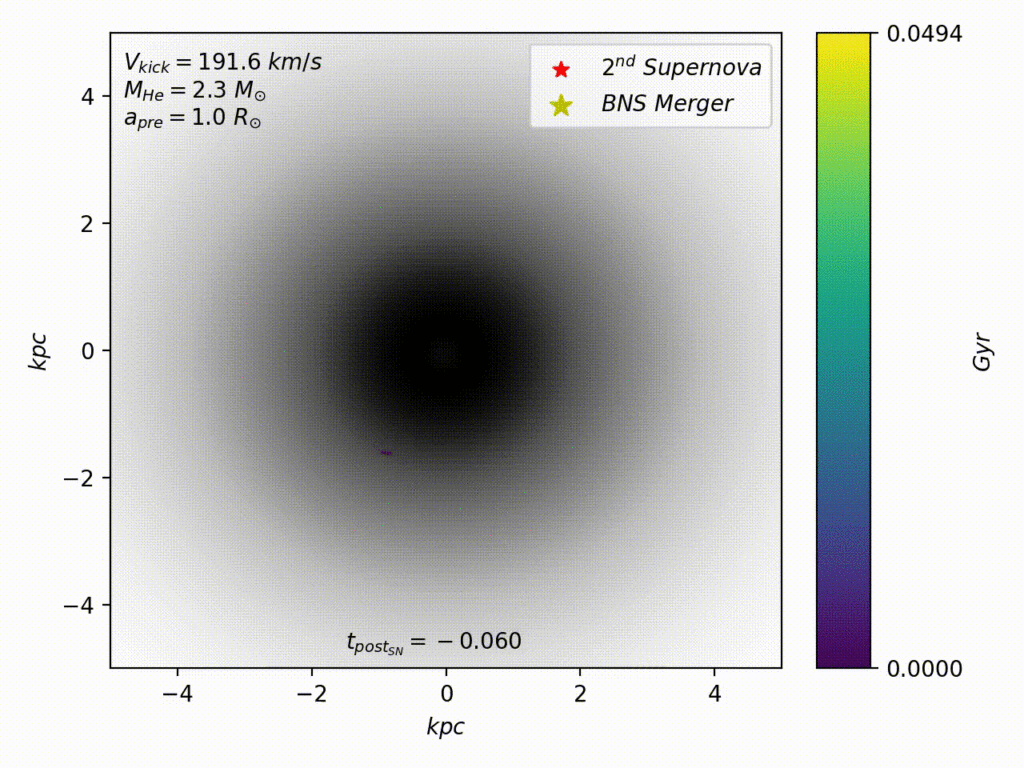
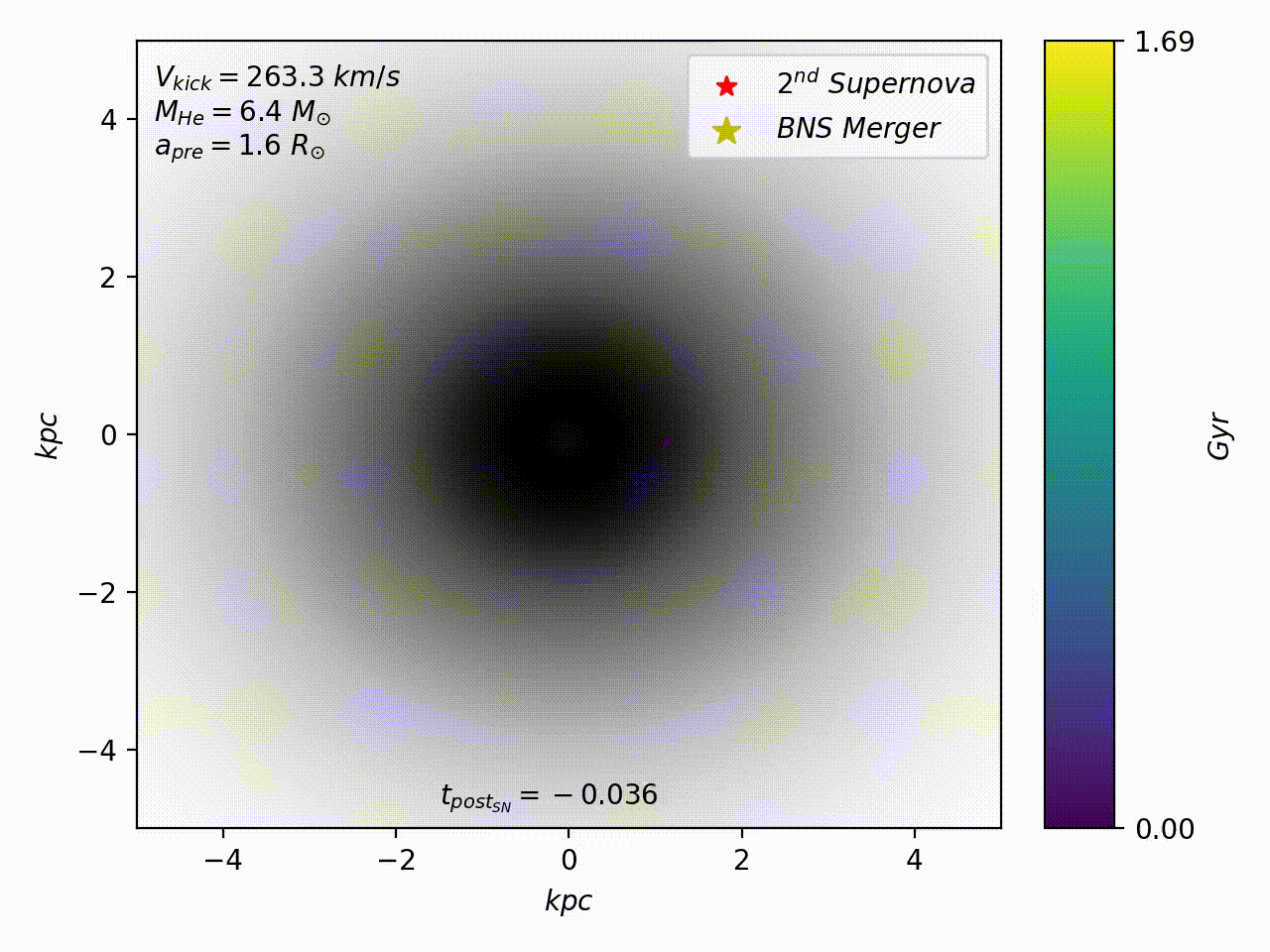
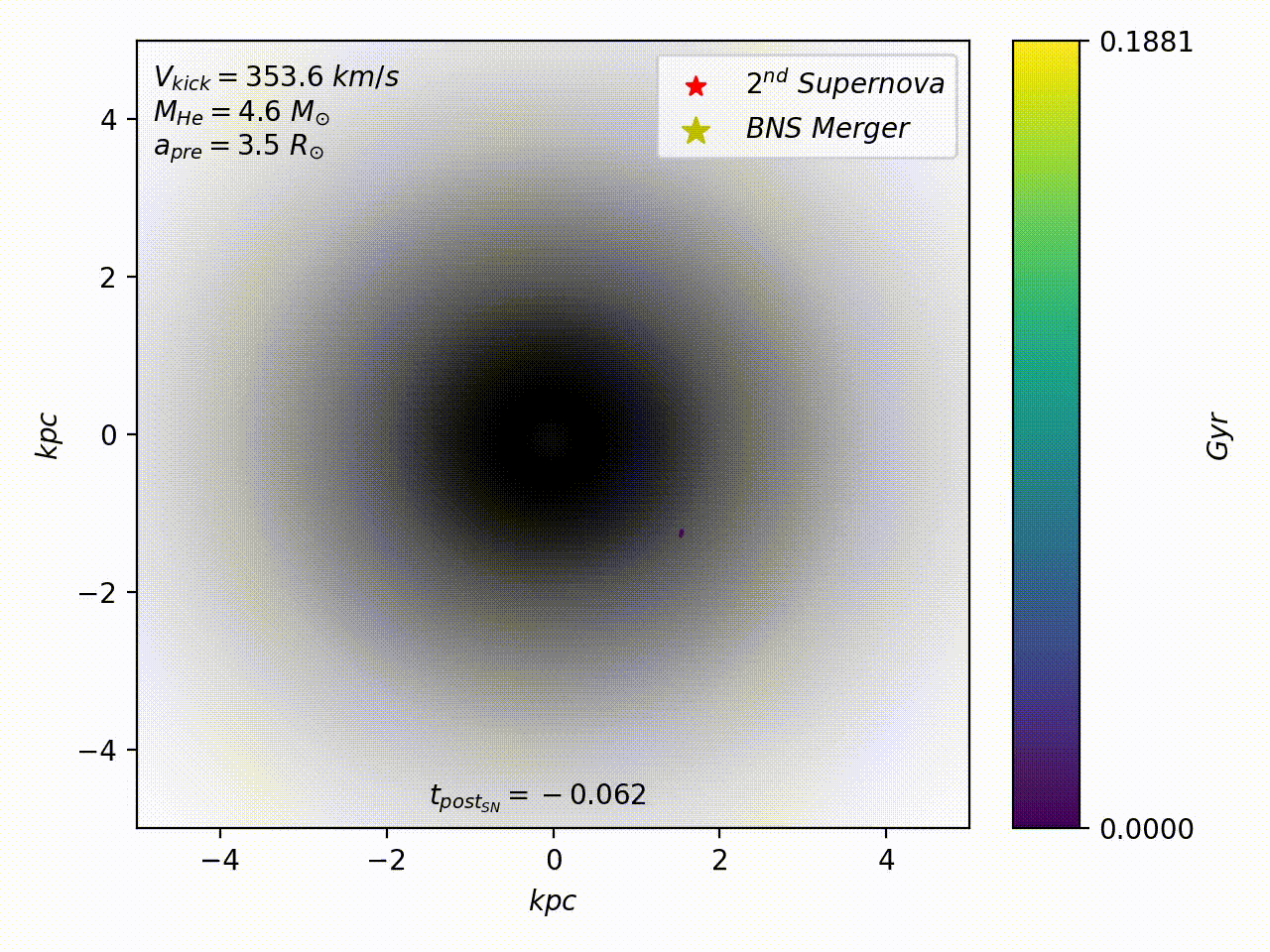
Example trajectories of simulated binaries neutron stars around a simulated galaxy, which would match our observations of GW170817. The line shows the path of the binary in the galaxy, the color coding is time until merger measured in gigayears; the red star marks the second supernova, the arrow shows the kick direction, and the yellow star marks the spot of the merger. Some trajectories are simple (like straight pots of a pool ball), others are more complicated (like bouncing the ball off several cushions before it reaches the pocket). See Figure 4 of the paper for more.
The results of our simulations is a set of Goldilocks parameters that are just right to explain our observations. At the time of the second supernova, the binary had an orbital size of around twice the radius of our Sun (which is pretty close for stars): smaller separations would mean that the binary merged too quickly, larger separations mean that the binary doesn’t merge at all. At the time of the second supernova, the binary was about two kiloparsec (7 thousand light-years or 100 billion times the radius of our Sun) from the center of the its home galaxy: more stars are formed closer to the center, but it’s also harder to escape from there out to where the counterpart was seen. The kick of the second supernova was around 300 kilometres per second: faster kicks are unlikely because they rip apart the binary. Finally, to give the right kick and the necessary change in the orbital separation, we second supernova probably came from a star that was around 3 times the mass of our Sun. These are our first results from a binary neutron star merger, as we collect more, we’ll be able to learn more how neutron stars are made, and exactly what happens during a supernova explosion.
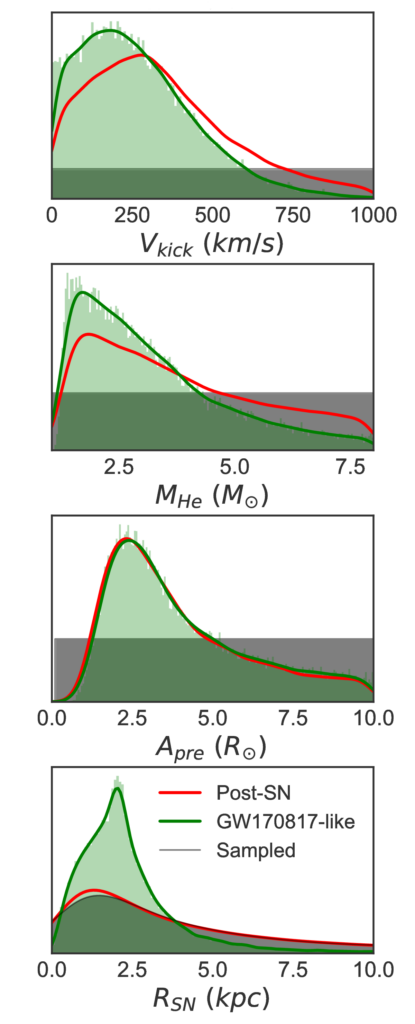
Inferred properties of the second supernova and the binary: the supernova kick velocity (top), the mass of the star pre-collapse, the orbital separation before the supernova, and the distance of the binary from the center of the galaxy (bottom). The solid band grey shows distribution of all the binaries we simulated (all the balls we hit). The red line shows the distribution of the all the binaries which go on to merge after the supernova (all the balls which go in a pocket). The green line shows the distribution for all the binaries that merge in a similar spot to GW170817 (all the balls which go in the right pocket). See Figure 5 of the paper for more details.
Find out more:
- Read the full article.