Discovering Gravitational Waves
It did not take long for the Advanced LIGO (aLIGO) detectors to make their mark on astronomy and astrophysics. The first gravitational wave source detected by the LIGO/Virgo Scientific Collaboration, named GW150914, has signaled the beginning of a new way we can learn about the Universe. The discovery itself of GW150914 is an awesome achievement, but the real fun begins as we try to decode what it has to tell us. To read more about the discovery of GW150914 visit the associated Science Summary Pages. Here we will summarize some of the first things we have learned about GW150914 in particular, and what it means for our broader understanding of astrophysics.
What Made the Gravitational Waves?
Once we have identified a gravitational wave in the data (more details here and here) supercomputers around the world start buzzing to determine what created the signal that we detect. We do this by trying out millions of combinations of different numbers (parameters) that describe a gravitational wave signal. The parameters that describe you might be height, age, hair and eye color, etc. The gravitational wave signal from GW150914 came from two dense objects spiraling in to one another and merging. The parameters that describe such an event are how massive the two objects are, how rapidly each object is spinning, where they are located, and how they are oriented towards us. By seeing which combinations of these parameters produce gravitational waves which match the data, we can measure different aspects of the true source. You can read about the details of GW150914 parameter estimation here.
The black holes in GW150914
From parameter estimation we now know that GW150914 came to us from the merger of two stellar mass black holes. The black holes that merged were each around 30 times the mass of the Sun, making them the largest stellar mass black holes ever observed. Stellar mass black holes form when a large star collapses in on itself, possibly triggering a supernova explosion. There are black holes millions of times more massive than the black holes which created GW150914 at the centers of galaxies, but they are not expected to be sources for aLIGO. The merging black holes we detected were likely located somewhere over the southern hemisphere of the Earth, and were measured to be about 1 billion light years away. Black hole mergers are incredibly violent events. Had all of the energy released by the merger come in the form of visible light, it would have briefly outshone the full moon despite being a billion light years away.
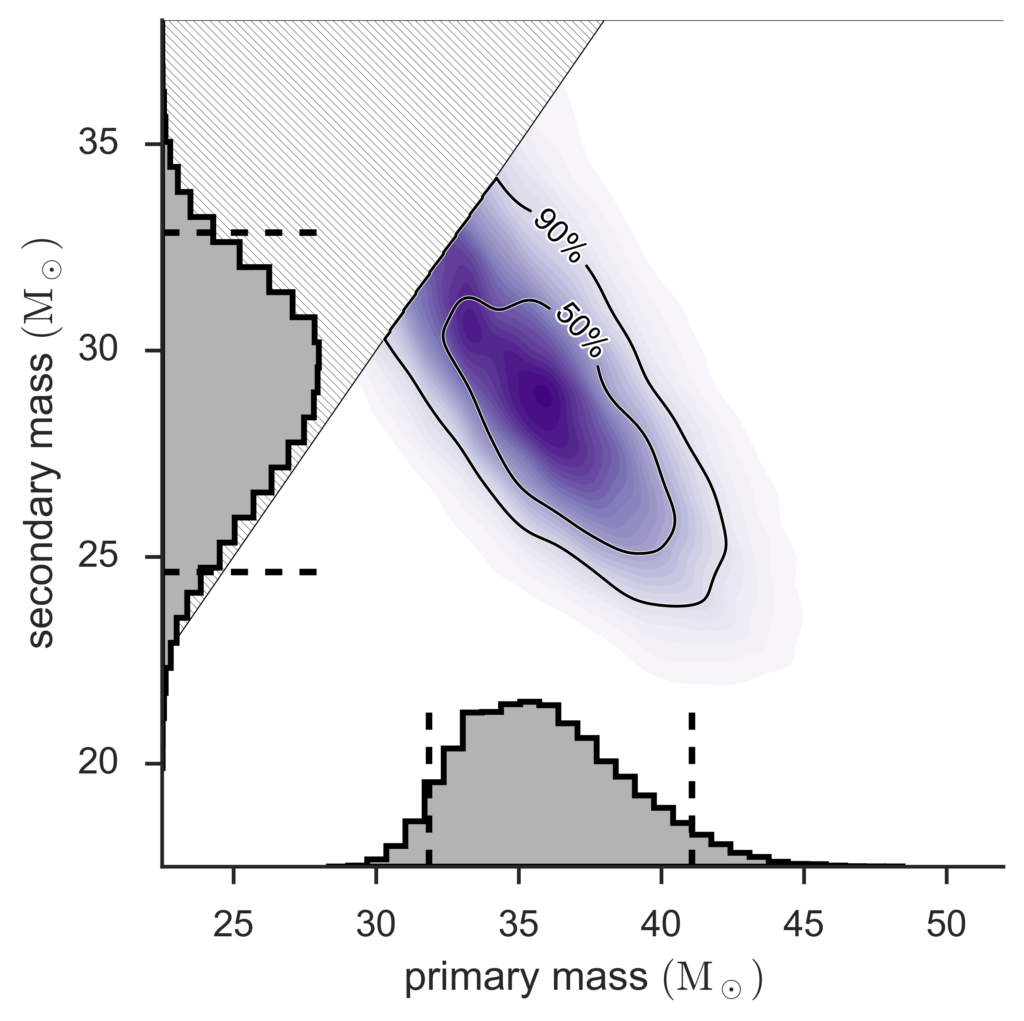
Figure 1: This image shows which combinations of the black hole masses are consistent with the data in units of the mass of the Sun. Places where the maps are darker have higher probability of being correct. We measure a 90% probability that GW150914 had masses inside of the black contour labeled “90%”.
Stellar mass black holes
Until the discovery of GW150914, our best way of studying stellar mass black holes was through the observation of X-ray binaries (XRBs), where material from a star orbiting a black hole is falling on to the black hole and, in doing so, emitting X-ray light. Currently there are 22 known XRBs where the mass of the black hole has been measured from optical light emitted by the binary; most have masses between 5 and 10 times the mass of the Sun, with a few up to 20 solar masses. All but three of the XRBs are within our own galaxy, making the black holes that created GW150914 the clear record holders in mass and distance from Earth. The relatively large masses of the black holes in GW150914 have interesting consequences for our understanding of black hole formation.
The formation of black holes from the collapse of stars is a very complex physical process and there are many unknown details that influence the end result, including the mass of the star before it collapses, how rapidly it is rotating, and details about how exactly the supernova explosion happens. The most sensitive feature in determining the mass of the remaining black hole is the metallicity of the star. To astronomers, “metallicity” refers to the fraction of a star made from elements that are heavier than Hydrogen (H) or Helium (He). Elements more massive than H and He are formed inside of stars or during supernova explosions. Just as the mass of objects are typically compared to the mass of the Sun, the metallicity of stars is compared to the solar metallicity. In order to form black holes as massive as those that made up GW150914, the stars that formed the black holes must have had around half the metallicity of the Sun, perhaps even as low as 1/4. Measurments like this will help teach us about the processes through which stars were converting H and He into heavier elements throughout cosmic time.
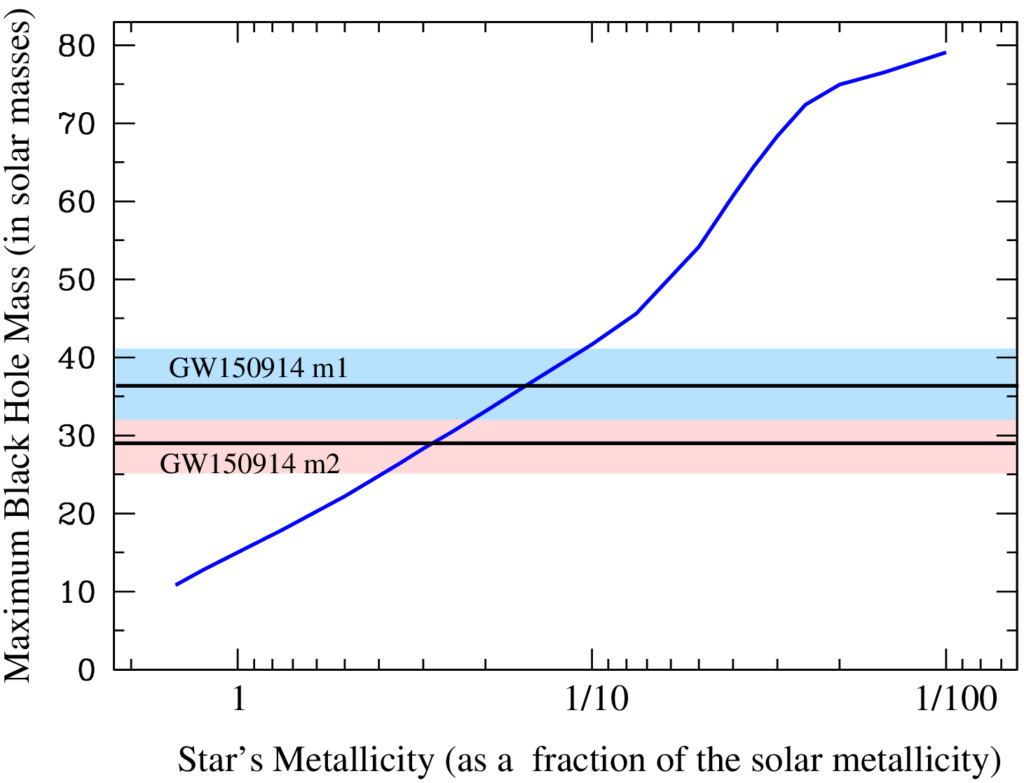
Figure 2: Maximum allowed black hole mass as a function of metallicity. The masses of GW150914 require metallicities well below the solar value (1 on this plot). Adapted from Belczynski et al. 2010, ApJ, 714, 1217.
Forming black hole pairs
While the individual masses of GW150914 tell us about the formation of black holes, what are the chances that two of them would merge with one another? Most massive stars are memebers of binary systems and most theoretical predictions have long suggested the existence of binary black hole systems, though none had ever been observed. The observation of GW150914 not only confirms that binary black holes exist, but that they are born sufficiently close together that they eventually merge, creating gravitational wave signals like the ones we detected. There are two main possibilities for binary black hole formation: Either a pair of stars orbiting one another each go supernova leaving behind black holes which eventually merge, or two separate black holes in a very dense cluster of stars come together and eventually merge. The tell-tale difference between the two, that we can measure from the gravitational wave signal, is the spin of the individual black holes.
Spinning black holes
Just as the Earth rotates about its axis as it orbits around the Sun, the two black holes will rotate as they orbit one another. And, just as Earth is tilted in its orbit relative to the Sun, the black holes may be tilted as well. How rapidly the individual black holes are revolving as they orbit one another, and how much they are tilted with respect to one another, is one of the first things we wanted to know about GW150914.
The Earth revolves once per day as it orbits the Sun. Black holes can, in principle, spin much faster than that but only up to a point. According to Einstein’s General Theory of Relativity there is a maximum rate at which a black hole can spin. The actual maximum spin of a black hole depends on its mass. We measuare the spin of black holes relative to their maximum allowed value. A black hole that has a spin of 1 would be spinning as fast as allowed by the laws of physics, a spin value of 0.2 means the black hole is spinning with 20% of the maximum value.
We are also very interested in the direction of the black holes’ spin. If you curl the fingers of your right hand around the equator of the Earth in the direction that it is turning your thumb points along the geographic north pole–that is the “direction” of Earth’s spin. You could do the same for each spinning black hole. We’ll call those directions “S1” and “S2”–one for each black hole. Now, if you curl your fingers in the direction that the black holes are orbiting one another your thumb will point in the orbital direction, which we’ll call “L.” If S1 and S2 point point away from L, the orbit precesses like a top wobbling around, causing L to move around as the black holes get closer. If S1 and S2 point in the same direction as L (or perfectly opposite), the orbit remains steady. Whether or not we can measure the orbital precession is an important clue into how the black holes formed in the first place. If there is not any precession it is more likely that the black holes formed together. If there is a lot of precession it is more likely that the black holes formed separately and before coming together. For GW150914 we could not definitively tell how large or small the individual spins were, or if they were causing the orbit to precess. We can tell that the total spin S1+S2 is likely small. The spin measurement for GW150914 suggests that the individual spins were either small, or they were pointed opposite from one another, cancelling each other’s effect. As aLIGO discovers more black hole mergers we will be watching closely for signs of precession to help unveil how binary black holes form in nature.
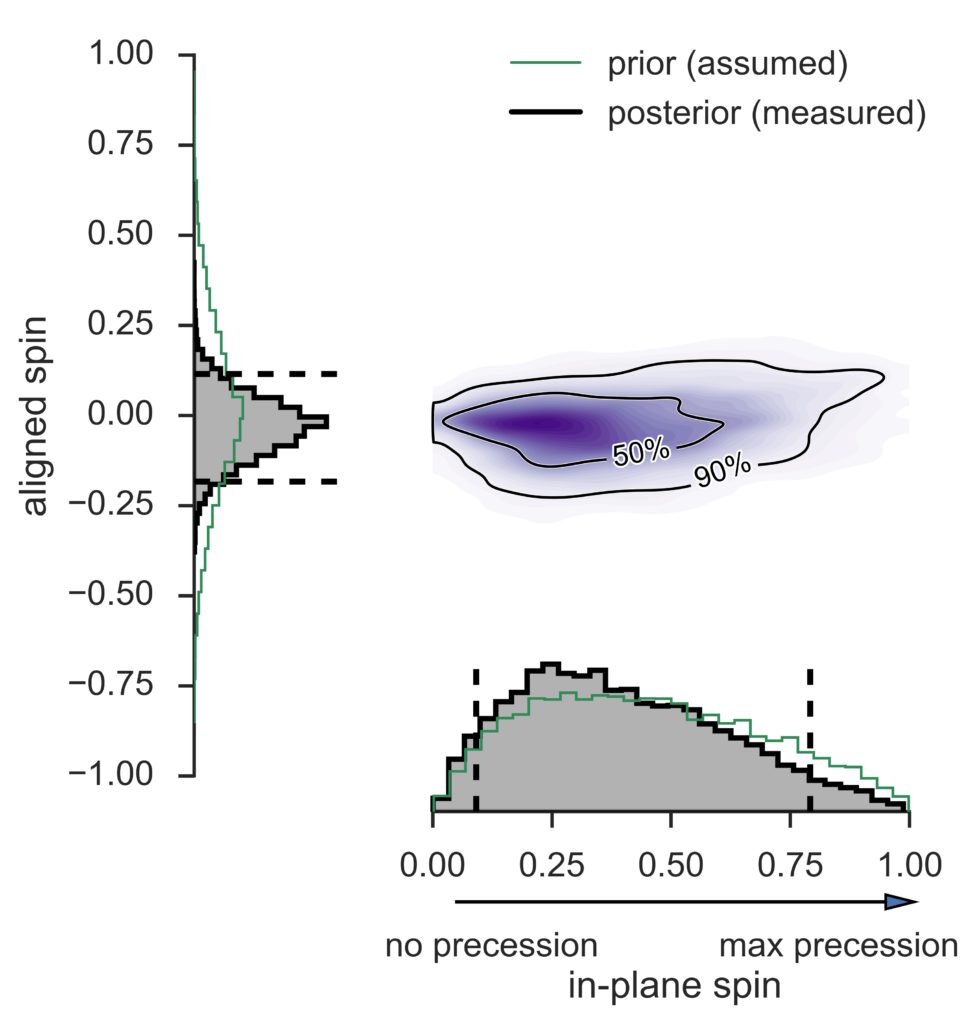
Figure 3: This image shows which combinations of the black hole spins are consistent with the data, in units of the maximum possible spin.
How Many More Will We Detect?
Right after the detection of GW150914 we started calculating how likely it was that we would find more during aLIGO’s first observing run (O1). Forecasting the chances for additional discoveries not only gives us something to look forward to, but is also a quantity that has implications for our understanding of how black hole mergers occur in nature. The number we aim to calculate is the rate of binary black hole mergers like GW150914 in the Universe. Our calculation of the black hole merger rate is described in detail here. There are two main ingredients to calculating the rate: The number of signals we have detected, and the sensitivity of our detectors. We measure the sensitivity as the volume of the Universe in which we could detect a black hole merger, times the amount of time we observed. We will refer to this quantity as the “space-time volume.” The amount of data analyzed so far is the equivalent of 16 days, although that is spread out over the first month of O1 because the detectors do not run constantly, and both have to be operating to detect gravitational waves. Once we calculate the rate of black hole mergers, we can use that number and our expectations for how sensitive aLIGO will be during the next science runs (O2 and O3, respectively) to make predictions for the number of binary black hole coalescences we will observe in the future. You can read more about future aLIGO observing campaigns here.
Counting black hole mergers
While GW150914 was the only event in the first 16 days of O1 that we are ready to claim as a “detection,” it was not the only piece of interesting data we have analyzed so far. Another candidate, LVT151012, was found in the data with a fairly low probability of being a false alarm (about 2%) which is enough to get our attention, but not enough to claim a detection. (LIGO detections are named “GW” followed by the date in YYMMDD format. LIGO/Virgo candidates start with a “LVT” for “LIGO-Virgo Trigger” follwed by the date. LVT151012 is an interesting candidate, but is not significant enough to “graduate” and become a “GW”). We have several even less significant events in the data, most likely just due to some disturbance at the detectors, which all (weakly) factor into the total number of possible black holes detected.
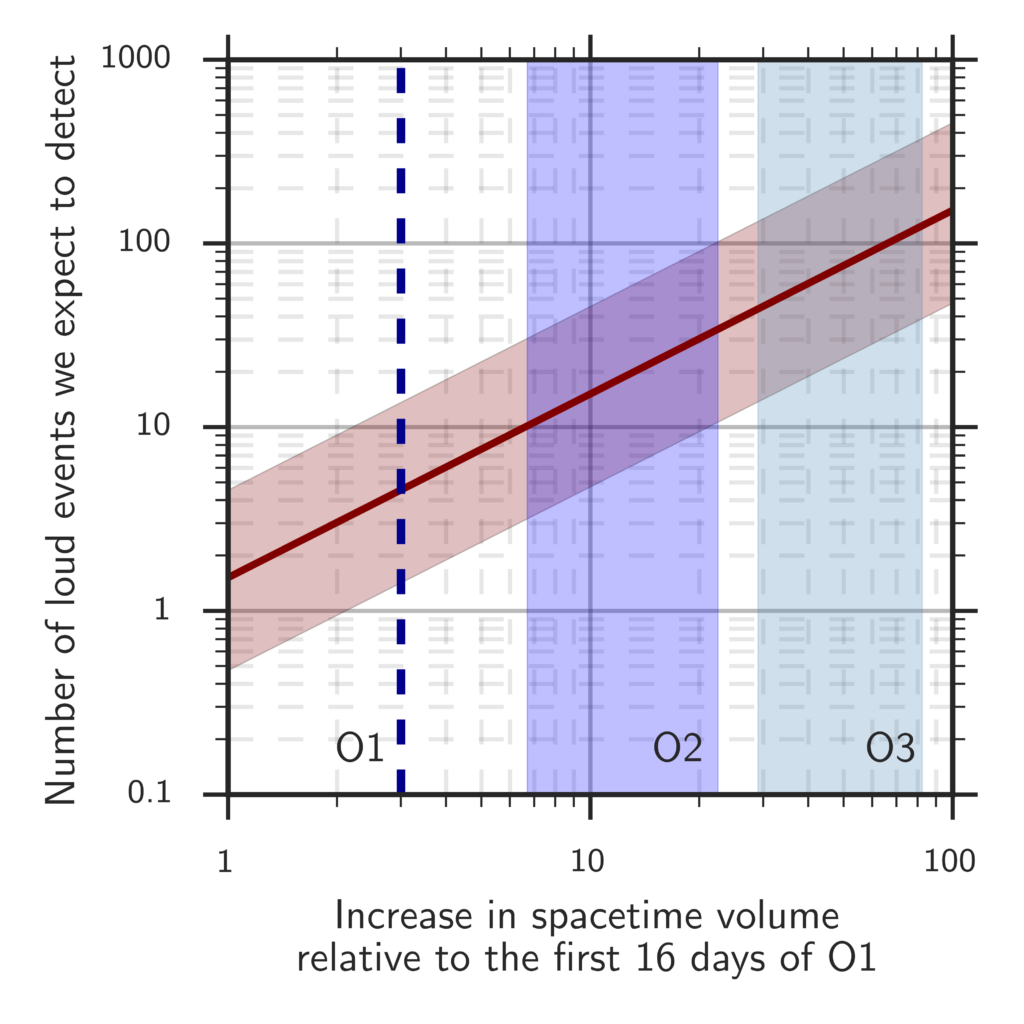
Figure 4: The maroon band shows the range of possible numbers of loud gravitational wave detections we expect to find as we increase the observed space-time-volume. The vertical bands show predictions for the space-time-volume observed (compared to the first 16 days of O1) by the next observing runs O2 and O3.
Measuring aLIGO’s sensitivity
To measure how sensitive aLIGO is to black hole mergers, we analyze data in which we have added simulated gravitational wave signals to see how far away from us it is possible for a source to be, for us to still be able to detect it. This is tricky business because the total mass of a binary black hole merger determines the maximum distance for which we can still notice it. With just one or two observed signals we still have very little guidance for the variety of possible mass combinations out there. To play it safe, we try several different hypothesis for different populations of black hole mergers and combine the results in the hopes that the truth lies somewhere within the different choices we explore. By combining the typical distances to which aLIGO can detect black hole mergers with the amount of time we have been observing we arrive at the space-time-volume of the observing campaign. With the count of black hole detections, and the surveyed space-time-volume we estimate that gravitational waves from black hole mergers somewhere in the Universe strike the Earth once every 15 minutes!
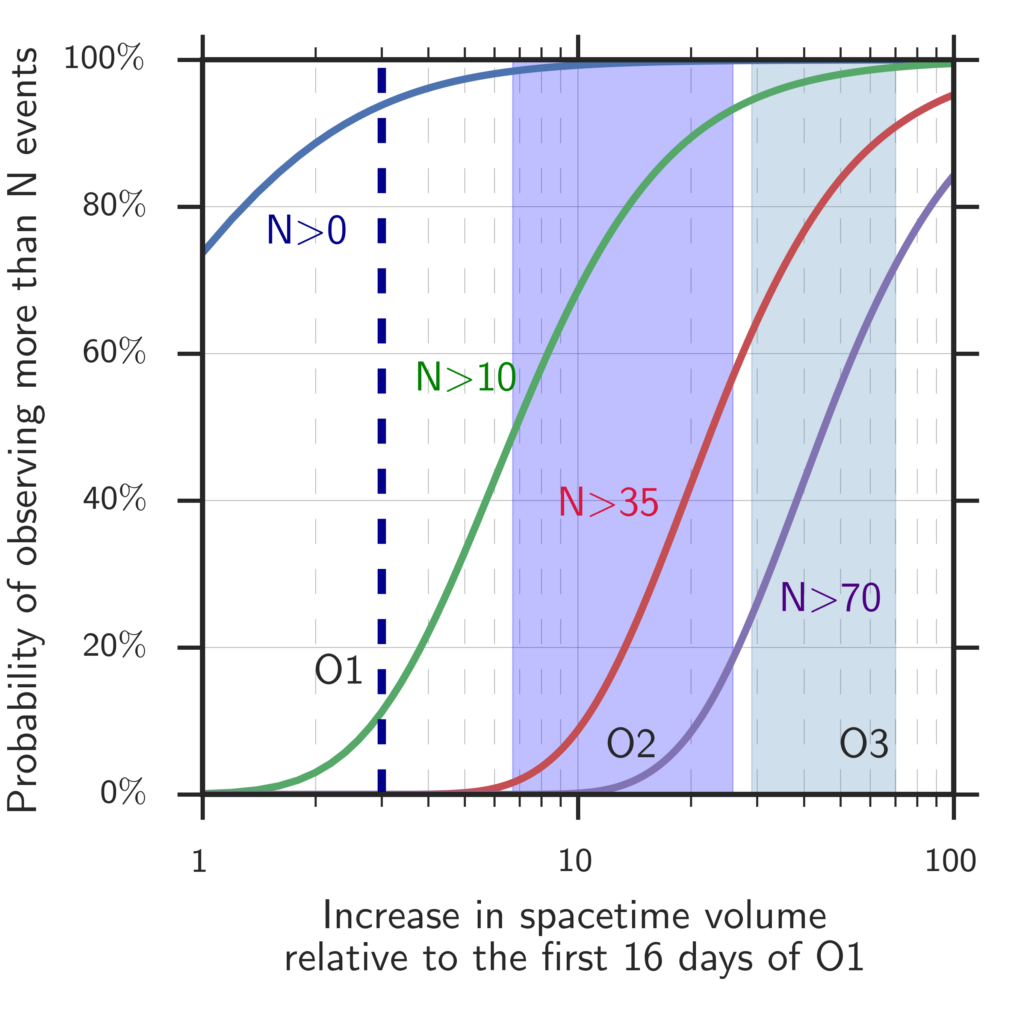
Figure 5: Different colored lines show the percent chance of detection greater than 0, 10, 35, and 70 loud black hole merger signals as we increase the observed space-time-volume. The vertical stripe shows the expected space-time-volume (relative to the first 16 days of O1) for the end of the first observing run. The vertical bands show predictions for the space-time-volume observed by the end of the next observing runs O2 and O3.
Looking ahead
With the number of black hole mergers counted in the data, and the sensitivity of aLIGO to the gravitational waves they produce, we can calculate the rate at which black holes merge in the Universe. The rate we estimate can then be combined with the expected sensitivity of the next aLIGO observing campaigns to give insight into how many detections we can look forward to. The numbers are very exciting! Figures 3 and 4 show the number of expected detections, and the probability of finding more than N detections, as we increase the space-time-volume surveyed by aLIGO. The space-time-volume of the different observing campaigns are marked on the figures. The forecasts are very uncertain because (a) with only a few detections we can not precisely pin down the rate and (b) the sensitivty of future observing runs depends on how well the upgrades go at the observatories. Taking these possibilities into account we expect between 3 and 90 significant black hole merger detections during the next observing run, and a nearly 100% chance of detecting at least 1. As we detect more gravitational wave signals we will learn new and finer details about the population of binary black holes in the universe. The detection of GW150914 is just one step into a brand new era of astronomy and astrophysics, and many more exciting discoveries are waiting for us.