There are many instruments which can detect gamma-rays operating in space, and they sometimes observe bright flashes which we call gamma-ray bursts (GRBs). Although they only last for a very short period of time, GRBs are the brightest electromagnetic events in the universe. The InterPlanetary Network (IPN) is a set of spacecraft with gamma-ray detectors which operate in the near solar system, from Mercury to Mars. Since these spacecraft are spread out in the nearby solar system, we are able to find out where on the sky the gamma-ray burst occurred using triangulation techniques because the burst will arrive at different spacecraft at different times. In general, GRBs are subdivided into two categories: long or short. The long GRBs have a burst duration (how long the flash lasts) longer than about 2 seconds, as well as having an energy spectrum dominated by relatively less energetic gamma rays (“softer”). Short GRBs have a burst duration shorter than 2 seconds and have a “harder” (more energetic) gamma-ray spectrum. Long GRBs are thought to be caused by the collapse of stars and make up the majority of the GRB population. We are less sure what causes short GRBs, although the current hypothesis is the collision of a neutron star with either another neutron star or a black hole. This interpretation was strengthened in 2013 with the observation of a kilonova associated with the short GRB 130603B.
The search for gravitational waves (GWs) offers an additional opportunity to study GRBs and to narrow down possible models for their origins. GWs are “ripples” in the curvature of spacetime, predicted by Albert Einstein’s General Theory of Relativity and produced by some of the most violent events in the cosmos, such as GRBs. For long GRBs, we search for any burst of gravitational waves that is detected in more than one detector, because with the collapse of a massive star, the signal might look different for each GRB. Therefore, we do not assume a specific signal shape for the GWs. For short GRBs, on the other hand, we are searching for a GW signal resulting from the collision and merger of a neutron star – neutron star (NSNS) binary, or a neutron star – black hole (NSBH) binary. These pairs of compact objects follow close orbits around each other; as they get closer together their orbital speed gets faster and faster, radiating increasing amounts of GWs and ending with a collision. We have well-understood theoretical models that predict the GW signal from such an event, so we can search for that signal in the GW data around the time when we know the short GRB occurred.
From 2005 – 2010, the LIGO and Virgo gravitational-wave detectors were operational near or at design sensitivity. This period included the fifth and sixth science runs (S5, S6) of the three LIGO detectors (two in Hanford, WA and one in Livingston, LA) and three science runs (VSR 1-3) of the Virgo detector in Cascina, Italy. During this time the IPN spacecraft detected over 600 GRBs. Since we want to have good quality GW data in multiple detectors, this narrowed down the number of GRBs to 223. Of these candidates, GW data around the times of 221 short and long GRBs were studied for evidence of any significant burst, with no prior assumption about what the signal should look like. (There were a further two short GRBs which occurred during these science runs for which there was not enough good-quality data to perform the unmodeled burst search). There were 27 short GRBs, out of the total of 223, on which we could also perform a search for NSNS or NSBH collisions.
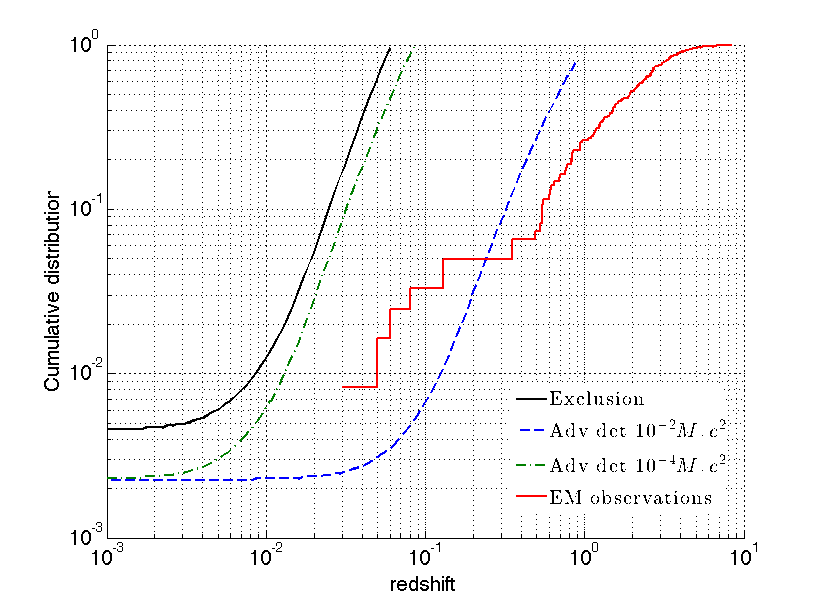
Exclusion distance for all 508 GRBs detected in the initial GW detector era using the unmodeled search for GW bursts. We extrapolate this for the advanced detectors by increasing the GRB population by a factor of two and increasing the redshift sensitivity by a factor of ten. We show two lines for different energies that the GRB could emit in GWs. If the higher energy emission is correct, then the advanced detectors should be able to detect some of the events which produce long GRBs.
We did not find any evidence for any kind of GW signals associated with these 223 GRBs. This is probably because the GRBs were too far away to be seen by our GW detectors. We combined this search with all of the previous GRB searches carried out with initial GW data, which together added up to 508 GRBs – including 69 short GRBs. Knowing how far our initial LIGO and Virgo detectors were able to “see” during this period means that we can place distance limits on the GRB population, which also gives us a better understanding of the population of astrophysical objects that produced GRBs. In particular, we can determine an “exclusion distance” beyond which we estimate (with a certain probability) the population of GRBs lies, given that we did not detect any GW signals.
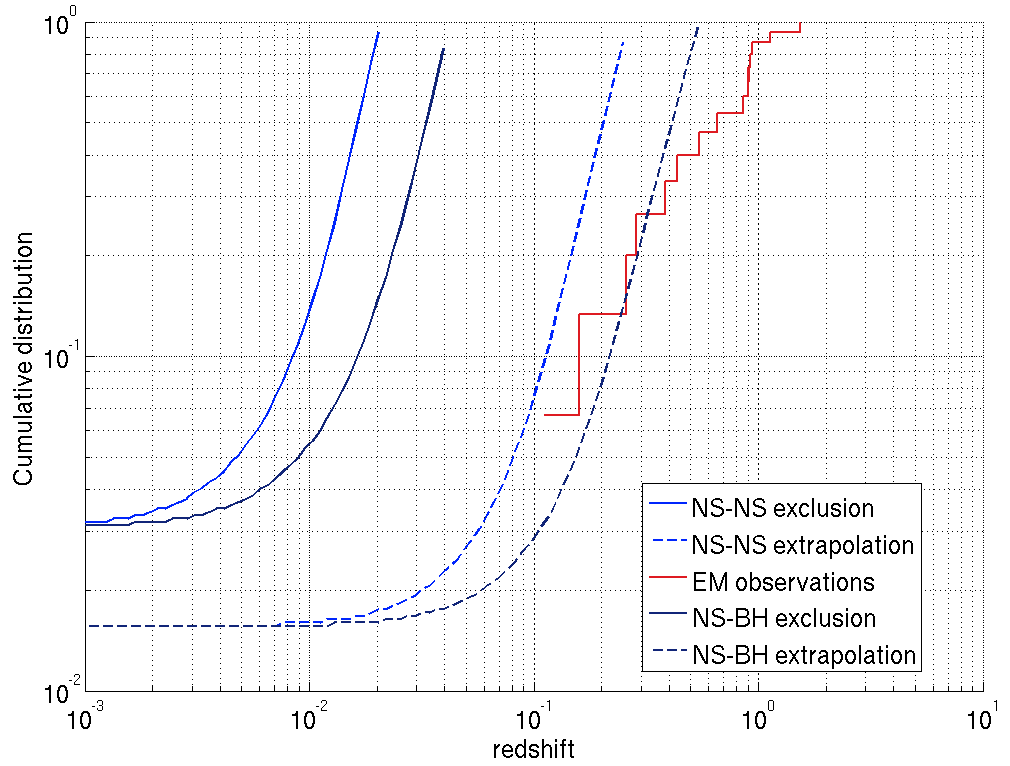
Exclusion distance for all 69 short GRBs detected in the initial GW detector era using the modeled search for colliding NSNS or NSBH systems. We extrapolate this for the advanced detectors by increasing the GRB population by a factor of two and increasing the redshift sensitivity by a factor of ten. We show two lines, one for the NSNS model and one for the NSBH model, as well as their extrapolations. These extrapolation lines lay very close to known GRB distances, making the likelihood of a joint GW/GRB detection likely in the advanced detector era.
Currently, the LIGO and Virgo GW detectors are being upgraded to Advanced LIGO and Advanced Virgo. These upgrades are expected to improve their sensitivity by a factor of 10 or more. This means that future searches will extend to distances at which GRBs have previously been observed. Additionally, the advanced LIGO and Virgo detectors will be operated for at least twice as long as the duration of the previous science runs. We therefore expect either to directly detect GWs associated with GRBs, or be able to eliminate and constrain various GRB models if no GWs are detected.
Read more:
- Freely readable preprint of the paper describing the analysis and results: http://arxiv.org/abs/1403.6639
- An introduction to gravitational waves from inspiraling binaries
- An introduction to gravitational-wave bursts