The first observation of a binary neutron star merger taught us a lot about the most extreme matter in the universe, but in the long run continuous gravitational waves from neutron stars (which need not be in binaries) can teach us more. Matter deep inside a neutron star is a hundred trillion times denser than rock, can have magnetic fields a quadrillion times stronger than on Earth, and may be made of stranger particles than any we see except as brief flashes in particle accelerators. Gravitational waves carry information about that matter more directly than electromagnetic waves (light, X-rays and so on) that only reach us from the surface of the star. Continuous waves in particular could tell us some properties of that matter which are hard to get from binary mergers, such as the elasticity of any solid phase or the viscosity of the fluid phase.
Young neutron stars are good places to look for continuous gravitational waves, since the supernova explosions that form them are violent, asymmetric events that might leave the new stars “roughed up” for some time. That is, the solid crust which neutron stars certainly have may not be smooth, and as a star rotates the roughness in the high density matter will emit gravitational waves. Also, electromagnetic observations of pulsars – neutron stars that appear as flashing dots in radio, X-rays, visible light, etc – tell us that many young ones are spinning down fast enough that they could be emitting strong gravitational waves. The youngest neutron stars (up to a few thousand years old) might also still have active r-modes, a type of wave in the fluid that makes up most of the star that can keep itself going through gravitational wave emission.
While a few of the youngest neutron stars are seen as pulsars, targeted by other LIGO and Virgo searches, more are seen as steadily glowing (in X-rays) points in supernova remnants. The precise positions obtained from X-ray observations (often with NASA’s Chandra satellite) give us enough information to search for continuous gravitational waves from these stars even though we don’t know the waves’ frequency and have to search over a band of frequencies (and different ways the frequency could go down over time).
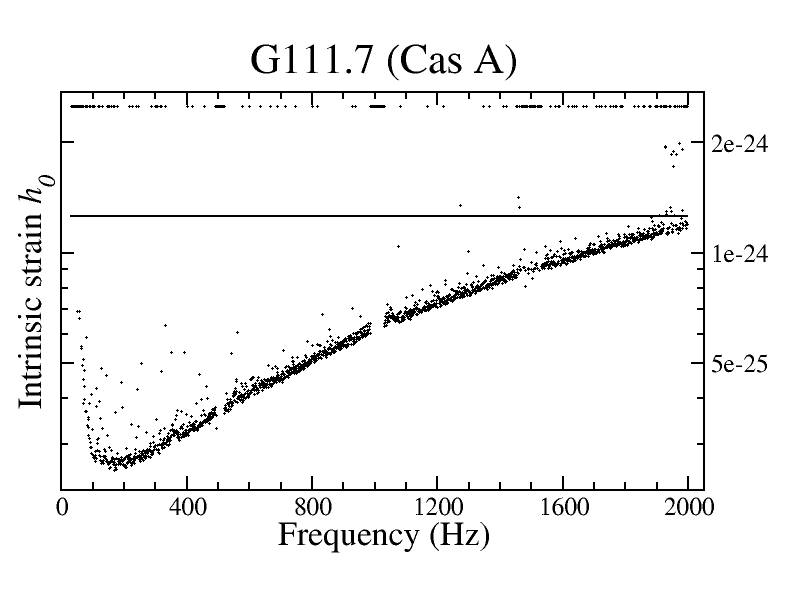
Upper limits on intrinsic strain (roughly a fractional change of LIGO arm lengths) for one of our targets, the supernova remnant Cas A, as a function of frequency in 1 Hz bands. The dots in a row near the top indicate bands omitted due to narrow-band instrumental noise. The solid line represents the strongest signal we could hope for based on energy conservation and the age and distance of the supernova remnant, and shows that we were sensitive to much weaker signals than that.
Using the same techniques we used to search Initial LIGO data for nine supernova remnants, we searched more sensitive Advanced LIGO data for continuous waves from young neutron stars in fifteen supernova remnants. These remnants included Cas A, which houses the youngest known neutron star, and Vela Junior, which may house the closest young neutron star. We also searched for Fomalhaut b, an object that might be an exoplanet or might be an old neutron star. This unusual object is worth checking because it is very close and therefore any gravitational waves would be relatively easy to detect.
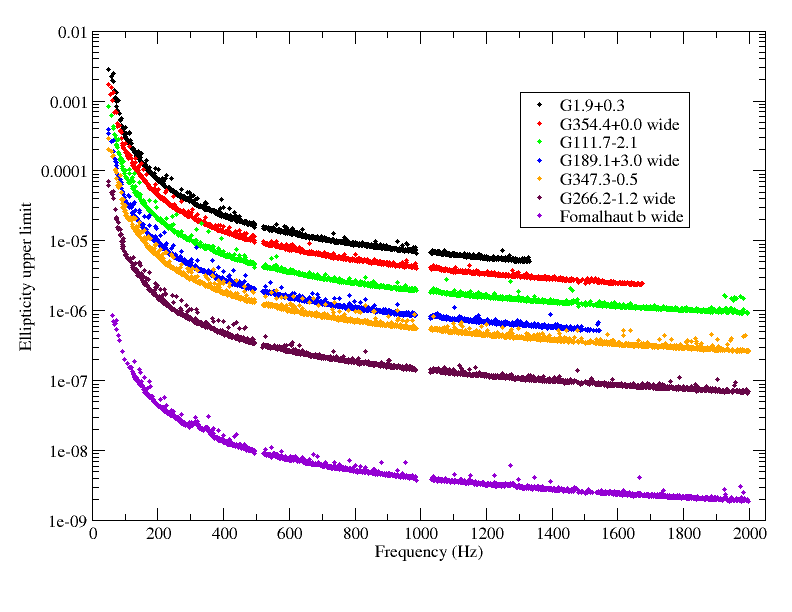
Like the previous figure, but showing upper limits on neutron star ellipticity (roughly the fractional height of a mountain in the solid crust).
We didn’t find any gravitational waves from any of these sixteen targets, so we set statistical upper limits on the signals that could have been buried in the detector noise. The strain upper limits depend on frequency mainly because the detector noise depends on frequency. The upper limits on ellipticity and r-mode amplitude, inferred mainly from independent estimates of distances to the neutron stars, depend more strongly on frequency because (all else being equal) a rapidly rotating star emits stronger gravitational waves than a slowly rotating one. On the right hand side of this page you can see plotted examples of the results. In the coming years, we’ll be able to look for fainter and fainter signals as the instrumental noise improves.
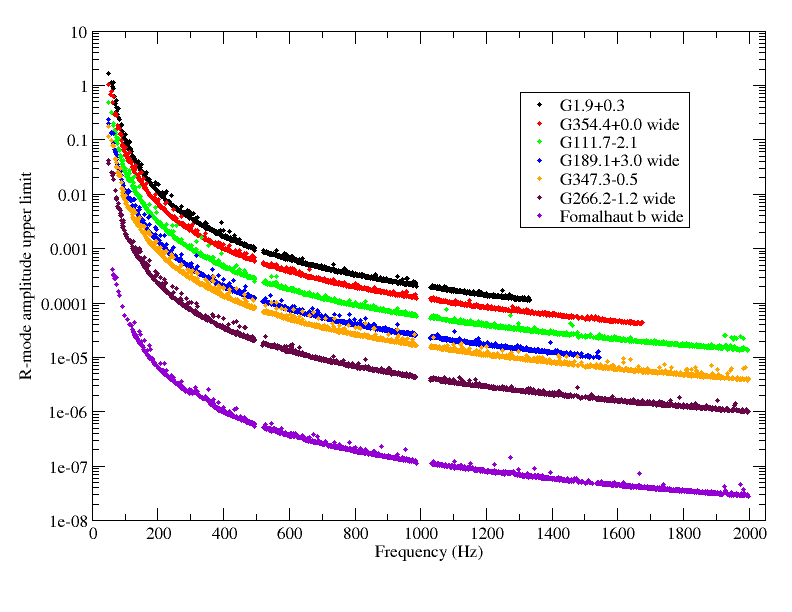
Like the previous figure, but showing upper limits on the amplitude of an r-mode (roughly the fractional change in velocity of the fluid part of a neutron star).
Read more
- Free preprint on arXiv.org
- Published version (The Astrophysical Journal, Volume 875, Number 2)
- Preprint of a similar search on earlier data
- Preprint of the first search of this type