There has been a lot of chatter in the news about the exciting findings of the BICEP2 experiment. But what do they mean? If the results are confirmed, BICEP2 will have been the first to observe the gravitational-wave imprint on the cosmic microwave background, a relic of the beginnings of the universe, and they will have found strong evidence for the theory of inflation, which describes the very first moments of the infant universe. They will have made the first observation of a gravitational-wave background.
A gravitational-wave background is something like a crowded room. You can hear the individual conversations of the loud people and the people closest to you, but otherwise the rest of the sounds blend together. You can tell that other conversations are happening, but you can’t tell them apart. The gravitational wave universe is the same way. We try to detect individual conversations (such as black hole collisions and gamma ray bursts, for example) as well as the overall “volume” of the room (like what we do here by measuring the amount of energy in the gravitational wave background).
There are many ways a background of gravitational waves might be made. For example, dense objects like neutron stars or black holes are predicted to make gravitational waves when they collide with each other. If there are enough of these collisions throughout the universe, the gravitational waves they produce may overlap to form a background where the individual collisions cannot be distinguished from one another. Or a gravitational-wave background might have been made in the inflationary era of the very early universe. When we look for the gravitational-wave background, we have the opportunity to learn about whatever contributes to it whether the contributions are from the first few instants of our universe or from much later on.
In this study, we were looking for direct evidence of a gravitational-wave background in the data taken with LIGO and Virgo observatories during 2009-2010. To look for the background, we compared data from different detectors to see if there were a common signal. This type search was also performed on earlier sets of data. As with many experiments, we were limited by the sensitivity of our detectors. This meant that, depending on what we found in the data, we could make one of two basic conclusions. Either we could measure the amount of energy in the gravitational-wave background if we made a detection or we could set a limit on the largest amount the energy of the gravitational-wave background could contain if we didn’t make a detection.
We searched for the gravitational-wave background in four frequency bands spanning 41.5-1726 Hz. This range of frequencies for sound waves is audible to the human ear (gravitational waves, however, do not make sound). BICEP2 observed at much much lower frequencies. Because our detectors have different sensitivity at different frequencies, having four frequency bands allowed us to make the most appropriate estimate of the gravitational-wave energy in each band. We found no evidence of a gravitational-wave background (our measurements were consistent with noise in the detectors) so we set upper limits on the amount of energy that could be in the background while still being consistent with our observations. The limits in these four bands are the lowest direct measurements to date on the background. In one band, the limit is a 38% improvement on previous results and in another band, the limit is improved by a factor of 2.5. The two remaining bands had never been investigated before so our limits are the first.
The fact that we did not detect a gravitational-wave background agrees with the predictions of most models. Of course, there’s always the possibility of seeing something that’s not predicted by a model, but we have ruled that out for anything that’s larger than our upper limits in the four frequency bands.
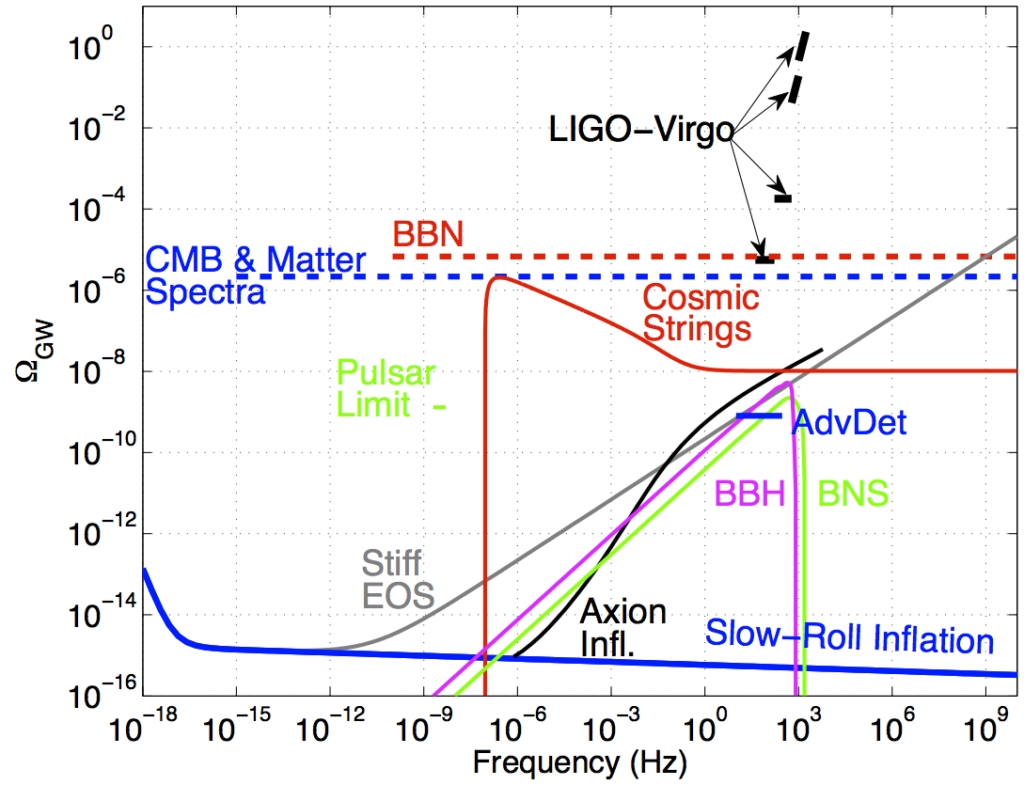
Our measurements of the amount of energy, ΩGW, contained in the gravitational-wave background (black, ‘LIGO-Virgo’). Since we found no evidence for a detection, we show upper limits on how large the gravitational-wave background could be. The plot also shows upper limits from some other measurements- ‘CMB & Matter Spectra’, ‘BBN’, and ‘Pulsar Limit’. These measurements were taken with a variety of different measurement techniques and study different frequency ranges. For comparison, ‘AdvDet’ shows the expected sensitivity of the advanced versions of LIGO and Virgo. The remaining lines show predictions from various models, some of which we may be able to test with the advanced LIGO and Virgo detectors and some of which will require building future detectors.
Once the advanced versions of LIGO and Virgo detectors are completed and start taking data, our sensitivity to the gravitational-wave background will improve substantially, potentially leading to a detection and the opportunity to learn about the different sources of the gravitational-wave background. To study the inflationary model claimed to be observed by BICEP2 at our frequency range will take a future generation of detectors. In the meantime, however, we will be able to probe many gravitational-wave models not accessible to BICEP2 with advanced LIGO and Virgo such as alternate theories of inflation and a background of black hole collisions.