What did we find?
The LIGO Scientific Collaboration and Virgo Collaboration can report that, on 25th April, 2019, gravitational waves were detected from the merger of two compact objects. Our collaborations designated this signal as GW190425. LIGO comprises of two gravitational-wave detectors, one in Hanford, Washington and the other in Livingston, Louisiana. At the time of GW190425, the LIGO-Hanford detector was temporarily offline, but the strong signal was detected in the LIGO-Livingston detector. The Virgo detector, located in Cascina, Italy, was also taking data. However, due to its lower sensitivity compared to LIGO and in particular because the source of GW190425 was likely in a region of the sky less visible for Virgo, the signal was only above the detection threshold in LIGO-Livingston. Nevertheless, Virgo data were useful in helping us to understand the parameters of GW190425’s source. We find the total mass of this binary to be between 3.3 and 3.7 times the mass of the Sun. Given this mass range, the most plausible explanation is that two neutron stars collided approximately 520 million light years away. The mass of this binary is significantly larger than any other known binary neutron star system.
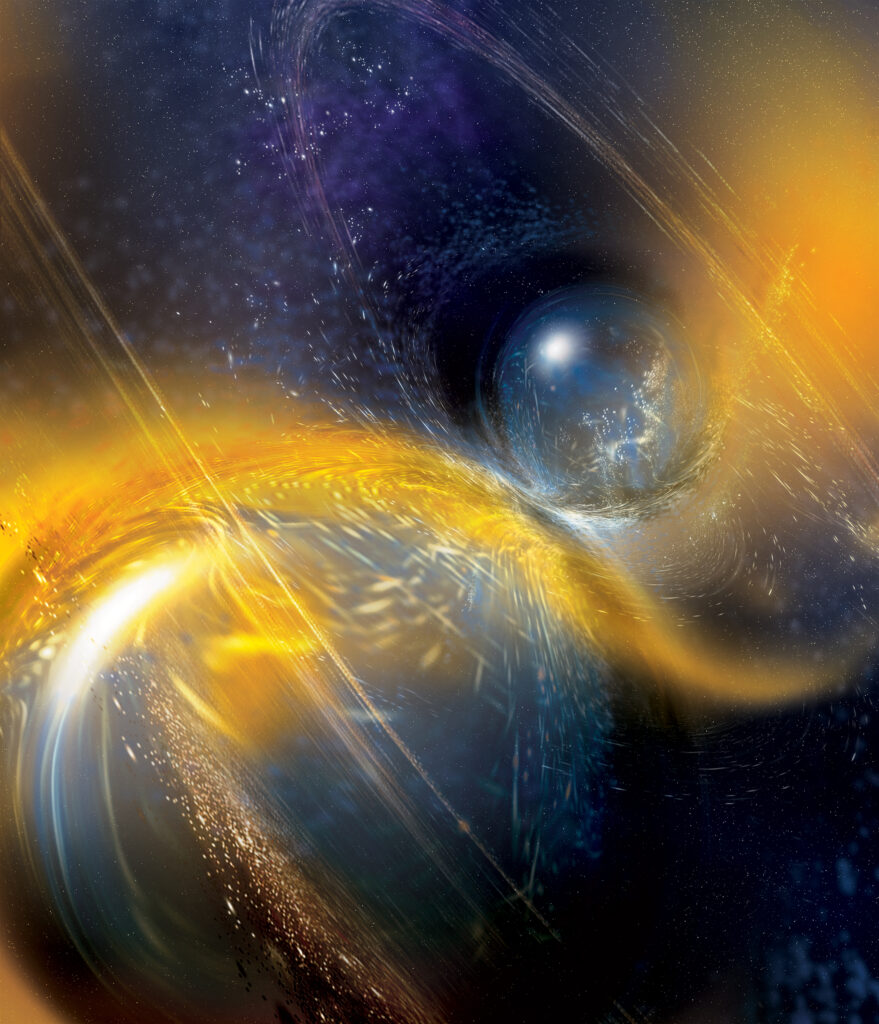
Figure 1: Artist’s impression of the binary neutron star merger producing GW190425. Credit: National Science Foundation/LIGO/Sonoma State University/A. Simonnet.
A little bit of background
GW190425 was detected in Advanced LIGO and Virgo’s third observing run known as O3, which started on April 1st 2019 and will finish on 30th April 2020. Prior to this observing run, there have been two other observing runs with the Advanced detectors, O1 (September 2015 – January 2016) and O2 (November 2016 – August 2017), see here for more details. Between observing runs the detectors are upgraded with new technologies to increase their sensitivity.
In O2, LIGO and Virgo made the first observation of gravitational waves from the inspiral of two neutron stars, known as GW170817. This collision of neutron stars also produced a counterpart seen across the electromagnetic spectrum. GW190425 is likely our second observation of a neutron star merger with gravitational waves. To date no electromagnetic counterpart or neutrino signal has been identified in association with GW190425. This is not surprising, however, given that the source was further away than GW170817 and so the anticipated electromagnetic signal would be weaker. But perhaps the biggest factor is because GW190425 was not well localized. In fact, we localized the source of GW190425 to a region covering about 16% of the entire sky. This is a huge area of sky for conventional telescopes to search!
How we know GW190425 is astrophysical
We have a number of searches which look for gravitational-wave signals from the merger of compact objects. They compare the observed data with theoretical signals predicted by General Relativity using a technique called matched filtering. Our search pipelines identified the GW190425 signal from the LIGO-Livingston data. The next step is to estimate how significant this event is, i.e. we want to know the rate at which we might expect such a signal to have occurred by chance due to the noise characteristics of the detector data. This quantity is known as the false alarm rate. To estimate this, we need to compare the strength of GW190425 with respect to a background distribution. A background was created by performing the search over 169.5 days of O1 and O2 and 50 days of O3, taken individually from LIGO-Livingston, LIGO-Hanford and Virgo. We found the false alarm rate for GW190425 to be one chance event in 69,000 years. Figure 2 shows that in the combined 219.5 days of background data, GW190425 clearly stands out from the background, in addition to the other confirmed detection of GW170817.
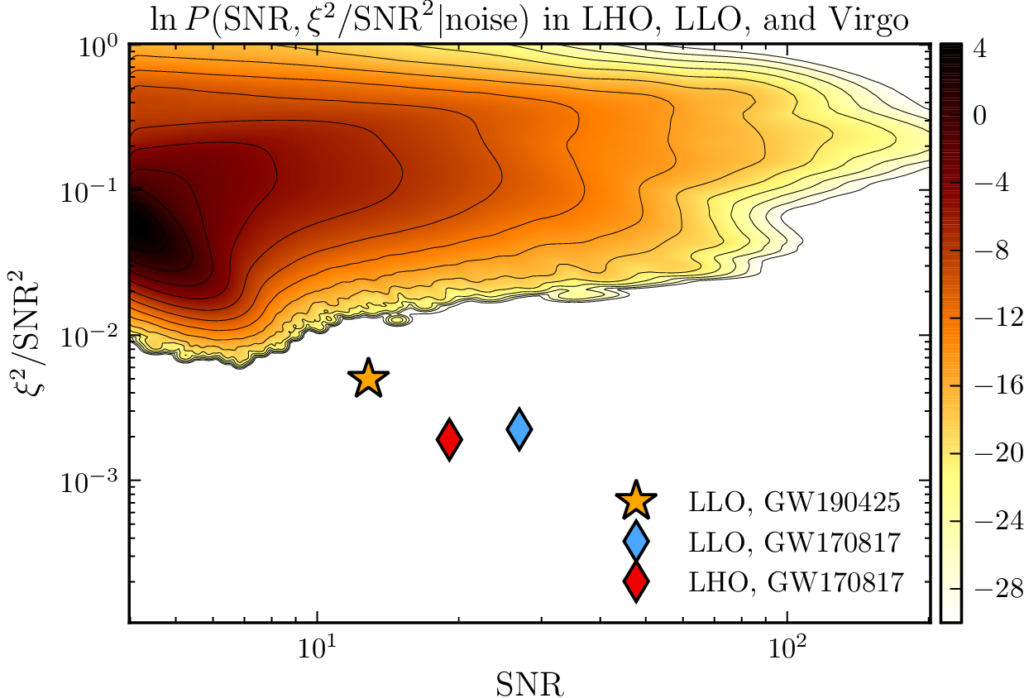
Figure 2: This figure shows the combined SNR–ξ2 noise probability function for LIGO-Hanford (LHO), LIGO-Livingston (LLO) and Virgo. The SNR (signal-to-noise ratio) quantifies how loud the signal is and ξ2 tests how well the time evolution of SNR matches that of a real compact binary signal. The figure shows the background in the region for binary neutron star signals. This background was constructed using 169.5 days from O1 and O2 and 50 days from O3. There is no background present at the position of GW190425 (gold star); it stands above the background. For comparison, the GW170817 signal recorded in the LIGO-Hanford and LIGO-Livingston detectors is shown by blue and red diamonds.
In addition to the searches, we also performed similar detection procedures on GW190425 as we have done for previous events. These checks investigate whether a rare instrumental noise transient at LIGO-Livingston could account for GW190425. We found no environmental or instrumental disturbances could account for GW190425.
Why is GW190425 so interesting?
We found that the mass of the heavier of the two compact objects is between 1.61 and 2.52 times the mass of the Sun, and the mass of the second object between 1.12 and 1.68 times the mass of the Sun. These masses are consistent with the masses measured from other neutron stars as well as what we might expect from supernova explosion simulations. The heaviest neutron star known from electromagnetic observations (PSR J0740+6620) is measured to be 2.05–2.24 times the mass of the Sun. For GW190425 we cannot rule out that one or both objects are black holes. However, the most straightforward interpretation is that these objects are indeed neutron stars. So, if this is the case, what can we infer about GW190425?
We find that in some ways GW190425 is not like other binary neutron stars in our Galaxy. While the mass of each neutron star is similar to those already known, the total mass is quite different. Figure 3 shows the total masses of ten galactic binary neutron star systems which are expected to merge within the lifetime of the Universe. A normal distribution has been fit to these 10 systems. We find the average galactic binary mass is about 2.69 times the mass of the Sun, while the mass of the GW190425 binary is about 3.4 times the mass of the Sun. In fact, it lies 5 standard deviations away from the Galactic mean. This suggests that GW190425 formed differently from these known Galactic binaries.
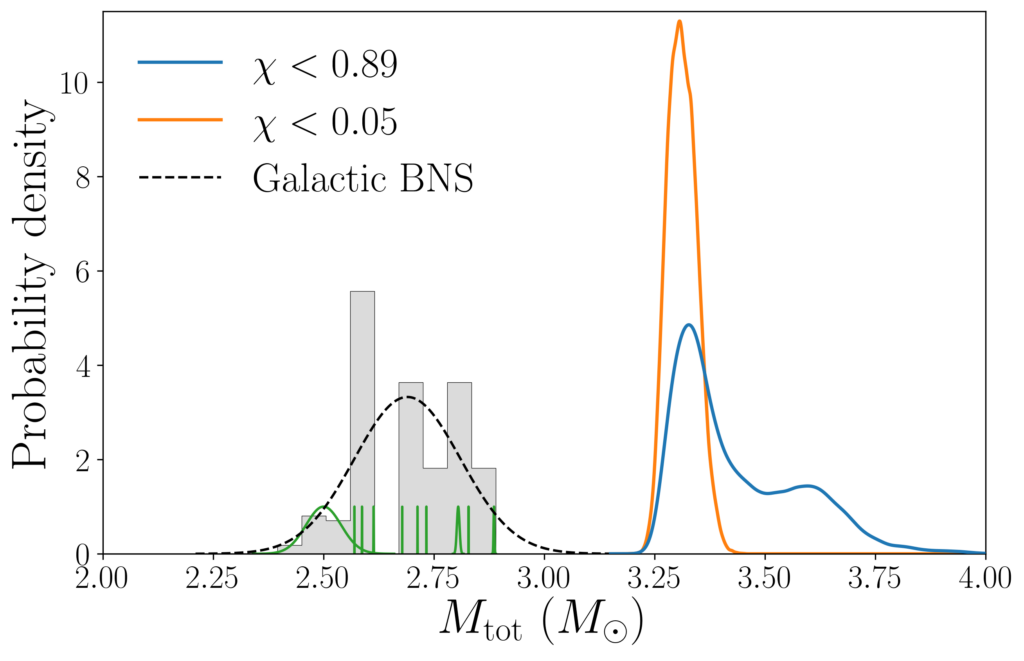
Figure 3: This figure shows the distribution of possible total masses for the GW190425 binary under different assumptions made about the individual spins of the two compact objects (blue and orange curves). Also shown are the total masses for 10 galactic binary neutron star systems that are expected to merge within the lifetime of the Universe. The distribution of the total masses of the Galactic binaries are fit using a normal distribution shown by the dashed black curve. The green curves are for individual Galactic binary neutron star systems rescaled to the same axis height of 1.
There are two ways in which we expect to form a binary with two neutron stars. One way is called the “common envelope isolated binary evolution channel”, where two neutron stars are formed when the two stars in a binary each undergo a supernova explosion but in isolation from other compact objects. The second way is called the “dynamical formation channel”. In this scenario, a binary already exists, which could contain two neutron stars or a neutron star and a main sequence star for example. Then another neutron star joins the two stars forming the binary and kicks out the lower mass star, leaving behind a binary containing two neutron stars. A dynamical origin is unlikely for GW190425 as this is not thought to contribute significantly to the merger rate of binary neutron stars. If the GW190425 binary formed in isolation, this may mean the neutron stars were born from low-metallicity stars. Or it may indicate that when the first supernova explosion happened and created the first neutron star in the binary, mass from the second star (which had not gone supernova yet) could have transferred on to the first neutron star and made it heavier. Either way, the discovery of GW190425 may suggest that there is a population of binary neutron star systems with sub-hour orbital periods which are not detectable by current electromagnetic surveys.
We also looked to see if we could tell how fast the neutron stars were rotating. Unfortunately, our results do not indicate what the spins of the neutron stars were. They are consistent with rotating like the two fastest spinning Galactic neutron star binaries that are expected to merge within the lifetime of the Universe, PSR J0737–3039A/B and PSR J1946+2052. This latter system contains a pulsar which rotates once every 17ms.
If we now take the discovery of GW190425 as a neutron star binary and combine this result with the one other binary neutron star we have observed (GW170817), we can estimate the number of neutron stars that collide in a volume of the universe every year. We find the rate of binary neutron star mergers to be between 250 and 2810 per gigaparsec cubed per year.
GW190425 is potentially the second observation of a binary neutron star, and it has given us more unique information about these strange objects.
Find out more:
- Visit our websites: ligo.org, www.virgo-gw.eu
- Read the LIGO press release about this discovery: ligo.caltech.edu/news/ligo20200106
- You can read the full scientific article here.