Why discovering “light” Black Holes would revolutionize Physics?
Advanced LIGO and Advanced Virgo have found a plethora of signals that are likely produced by the gravitational waves emitted during the coalescence of binaries of compact objects (black holes and neutron stars). Most of the black holes routinely observed in this way are usually between ten and one hundred times the mass of our Sun. However, the LIGO–Virgo–KAGRA Collaboration also looks for less massive compact objects. In this publication, we present such an endeavor, using data collected by the interferometers from 1 November 2019 to 27 March 2020 spanning the second half of the third observing run (O3b).
Detecting even a single black hole less massive than the Sun would have groundbreaking implications for our understanding of the Universe. The reason is the following: no known astrophysical process could make the core of a star collapse into a black hole with a mass below one solar mass (the mass of our Sun). Furthermore, there is also no firm observation of neutron stars lighter than the Sun (neutron stars could possibly be confused with black holes in gravitational-wave signals). Such black holes, which we call subsolar mass black holes, would therefore be the result of a novel phenomenon in the Universe.
One possibility that has recently attracted lots of attention is that such black holes, and possibly some black holes already detected by LIGO and Virgo, could have formed a fraction of a millisecond after the Big Bang due to the collapse of pre-existing lumps in the primordial plasma. These are called primordial black holes and their reality was conjectured in the 1970s. Among others, the existence of such primordial black holes could explain some or all of the mysterious dark matter depending on the primordial black hole distribution and range of masses considered. More massive primordial black holes could also be at the origin of the supermassive black holes observed in the center of galaxies.
However, primordial black holes are not the only candidates for subsolar mass black holes. If dark matter is made of a new particle interacting with Standard Model particles, it can be captured by compact objects such as neutron stars which would then be transmuted into black holes. If dark matter were to be composed of various ‘dark’ particles that interact with each other so as to allow for sufficient cooling, dark matter black holes could have formed via the collapse of dark matter clouds.
Methodology: How to detect light black holes in gravitational-wave observatories
As in the standard gravitational-wave searches on which the GWTC-3 is based, several matched-filter analysis pipelines are employed to identify subsolar mass candidates. Specifically, the three pipelines participating in this search make use of templates that represent our best description of the inspiral part of a binary black hole coalescence. These are compared to the signal in the detectors, enabling us to find gravitational waves buried in the noise. Using three pipelines allowed us to cross-check and validate the obtained results. The main difference with the GWTC-3 search lies in the parameter space probed. In this subsolar search, the mass of the smallest black hole in the binary goes from 0.2 to 1 solar mass, while the mass of the largest black hole can range from 0.2 to 10 solar masses. Typically their signal is longer and fainter than the one of other observed, more massive, black hole coalescences. For instance, a binary black hole signal with both masses equal to that of our Sun lasts 280 seconds in the detectors’ sensitive band. Comparing this to the 0.2 second signal of the first observed black hole coalescence, GW150914, gives an idea of the much larger computing resources needed for their search!
Did we discover something?
To quantify the significance of a match between a template and the data, the aforementioned pipelines estimate how many times per year, on average, the detector noise is expected to mimic something as signal-like as what is observed. This is called the false alarm rate (FAR). We find that no candidate is significant enough to be considered a discovery, the best one has a FAR of 1 per 5 years. For comparison, GW150914, which was used to claim the first direct detection of gravitational waves, had a FAR of less than 1 per 200000 years.
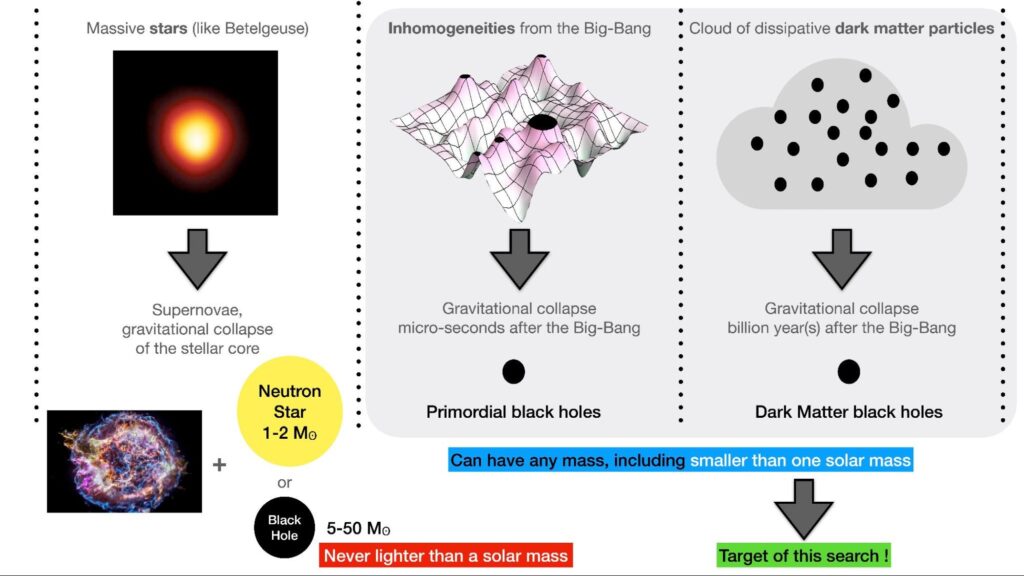
Figure 1: Sketch of the different pathways to form the compact objects that can be observed by LIGO-Virgo-KAGRA. Besides neutron stars and black holes formed by stellar evolution, there are hypothetical primordial or dark matter black holes that could exist in the Universe and be less massive than our Sun. Click on the image for a larger version.
Injections & constraints
In Science, much information can be extracted even if we do not make a detection. Considering the astrophysical range of the detectors and the duration of observations, the absence of any firm detection can be used to put upper limits on the merger rate density of subsolar mass objects. Since the search sensitivity is primarily a function of chirp mass, we show in Figure 2, as a function of this parameter, the values of the merger rate density excluded by the absence of detections. The constraints were derived using the whole O3 run, getting a result twice as strict when compared to previous searches. As we can see, the limit on the maximum merger rate allowed is consistent amongst search pipelines. For example, if we look at the binaries with a chirp mass of about 1.5 solar masses, we determine that there have to be less than 300 mergers per cubic gigaparsec per year. This is still about 10 times larger than the merger rate as measured from the binary black holes of GWTC-3, which are heavier than a solar mass.
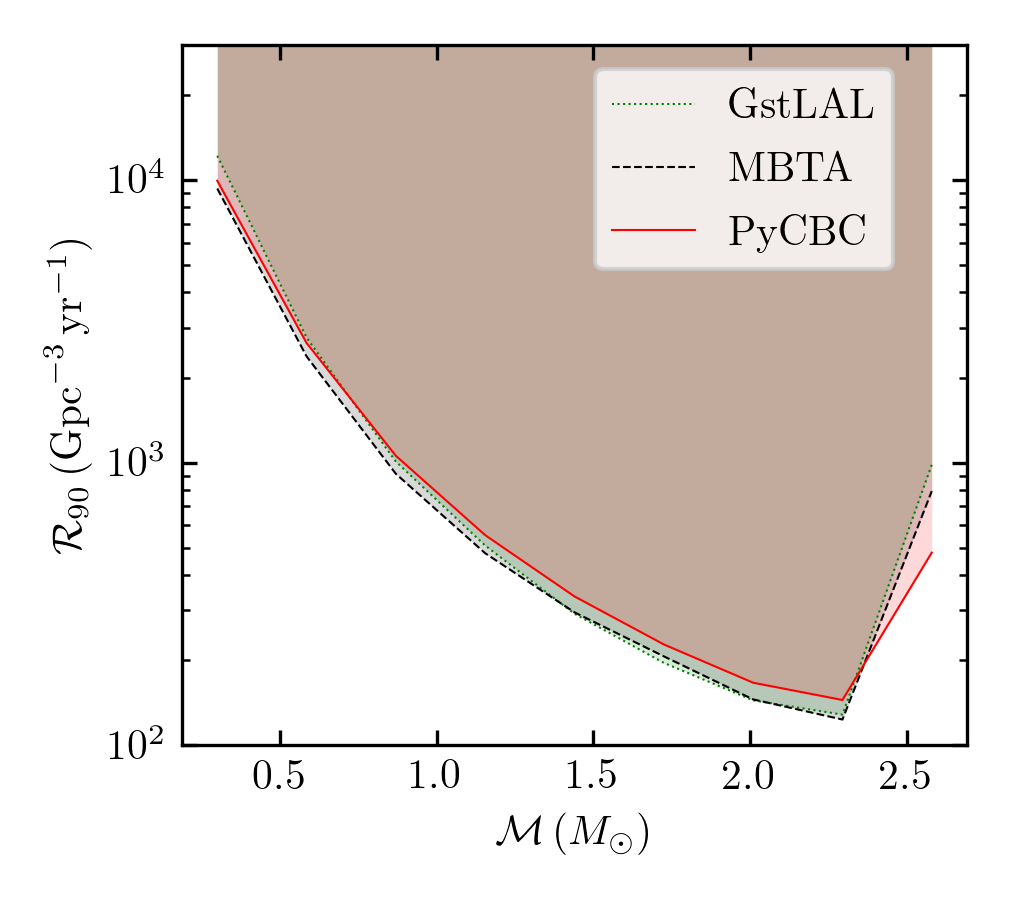
Figure 2: Merger rate upper limits (in units of the number of mergers per year per cubic gigaparsec) as a function of binary chirp mass for the three different search pipelines used in the analysis (GstLAL, MBTA and PyCBC). The lines represent the merger rate upper limits and the coloured zones mark the values of the merger rates that are excluded because our search did not make any detections.
These merger rate limits translate into constraints on any model’s parameters that predict subsolar mass objects’ mergers. Two scenarios have been considered: primordial black holes and black holes made of dark matter particles.
In the case of primordial black holes, we consider the simplest model in which all black holes have the same mass and are randomly distributed in space, with the binary formation happening in the very early universe, just after their formation. We include physical effects that were not considered in previous searches, like the breaking of binaries by other primordial black holes. In the range of masses to which our analysis is sensitive, between 0.2 and 1 solar masses, we are able to exclude for the first time that primordial black holes described by this model constitute all the dark matter in the Universe. However, we cannot significantly constrain models of dark matter made of primordial black holes with a wide distribution of masses.
We also obtained improved limits on the dark matter black holes. We find that no more than about a thousandth of a percent of all the dark matter could have collapsed into these objects.
Prospects for O4
The LIGO and Virgo detectors are now offline for improvements before the upcoming fourth observing run, currently set to get underway in 2023. The KAGRA detector in Japan will also join the O4 run. The network’s sensitivity will increase significantly, boosting the chances of detecting such an unprecedented event or further improving the constraints on their abundance.
Find out more
- Visit our websites:
- Read a free preprint of the full scientific article here or on arXiv.org