What makes eccentric binary black holes special
The LIGO and Virgo gravitational-wave observatories have discovered nearly 100 compact binary mergers so far. Most of these are binary black hole mergers. The astrophysical origin of these violent events still remains a mystery.
Gravitational-wave signals carry information about various properties of binary black holes. One such property is the orbital eccentricity of the binary: the more elliptical the black holes’ orbits are, the more eccentric the binary is said to be. This obscure property of the binary actually reveals key details about the origin of merging black holes.
Most black holes in the Universe are formed when massive stars die. How two black holes form a binary and eventually merge can happen in several different ways. For example, two stars that orbit each other can naturally end up forming a black hole binary. At the same time, two black holes that are initially far apart can come together by chance in places in the Universe where a large number of black holes reside in a small volume, such as in the centers of galaxies.
What does this have to do with eccentricity? When the black holes orbit each other, they emit gravitational waves — undulations in the fabric of space-time — which makes them orbit ever closer and eventually merge, since the waves carry away orbital energy and angular momentum from the system. At the same time, gravitational-wave emission also acts to make the binary’s orbit more circular. Binaries that orbit each other for a long time (say, a billion years), end up having no eccentricity.
If we detect a binary that has an eccentric orbit, it can mean one of two things: the black holes lived in a binary only for a short time before merger so that gravitational waves didn’t have time to make their orbit circular. This can happen if the black holes meet by chance and their orbit is small from the beginning. Second, something other than the two black holes could have interfered with the orbit to make it more eccentric. This could be a third nearby object, such as another black hole or a star, or even gas that may surround the black holes.
Detecting eccentricity therefore is a forensic tool that allows us to probe the black hole’s past.
In this analysis, we present a search dedicated to look for eccentric binary black hole (eBBH) coalescences in the third observing run by LIGO and Virgo. We also determine the astrophysical implications of the search results.
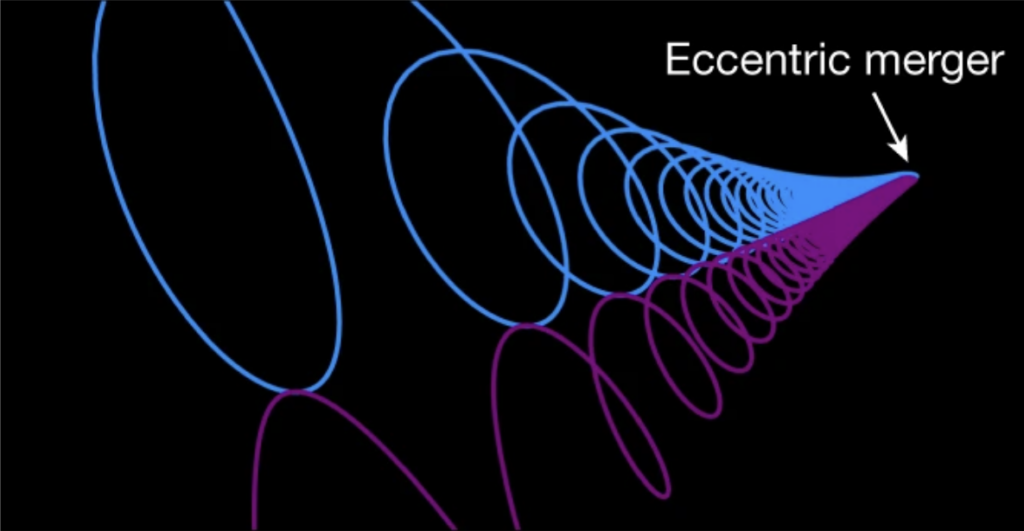
Figure 1: Illustration of an eccentric binary black hole merger in the AGN disk. A high fraction of binary black holes in the AGN disk are expected to have eccentric orbits due to interactions with other black holes in the disk [Credit: Samsing, J et al. Nature 603, 237–240 (2022).
How we looked for them
There are various challenges that we encounter when looking for eBBH systems. Due to the fact that orbital eccentricity is constantly evolving as a result of gravitational-wave emission, it is challenging to define this quantity as well as model gravitational-wave signals coming from eccentric binaries. Currently, no comprehensive eBBH template bank exists, making model-based searches unsuitable to look for eBBHs. In our analysis, we therefore employed the minimally-modeled search algorithm coherent WaveBurst to search for eBBH signals. This search algorithm looks for coincident deviations from the expected detector noise at two or more of the LIGO and Virgo detectors with minimal assumptions about the signal morphology. We optimized this algorithm to be more sensitive to eccentric binaries using state-of-the-art numerical relativity simulations from the Simulating eXtreme Spacetimes (SXS) Collaboration. We carried out the eBBH-optimized search over the third observing run of the LIGO and Virgo detectors.
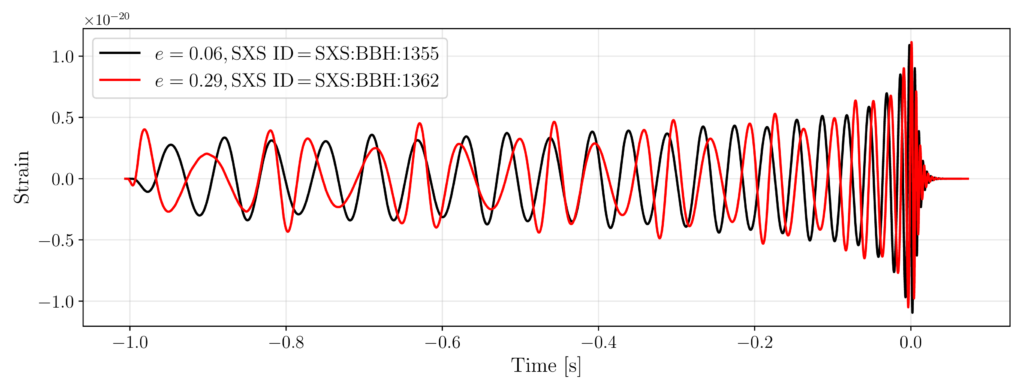
Figure 2: (Figure 1 from the paper) This figure shows simulated eccentric waveforms for two different eccentricities for an equal mass binary system with total source mass of 90 M☉ (where M☉ denotes the Sun’s mass) at a distance of 100 Mpc, which is about 325 million light-years. The red waveform depicts a binary characterized by a notably higher eccentric orbit compared to the black waveform. The simulations start at an orbital separation that translates to an emission frequency flow=15 Hz.
What our search found
The eBBH search recovered one new candidate that has not been previously reported by searches targeting quasicircular binary black holes. We performed various follow-up analyses to better understand the most significant new candidate. As a first step, we ascertained that this candidate did not occur at the time of any known instrumental or environmental artifacts. Following this, we estimated the properties of this candidate using a quasicircular waveform model. This analysis indicated that if real, the new candidate was most consistent with a signal from a high-mass binary black hole coalescence (assuming it originated from a system with low to moderate orbital eccentricity). Through these investigations, we were unable to conclusively determine if the event was of astrophysical origin or if it was a result of a noise fluctuation intrinsic to the detectors. Due to lack of conclusive evidence confirming whether the detected event was of astrophysical, we proceeded under the assumption that the search did not detect any eccentric signals for the remainder of the analysis.
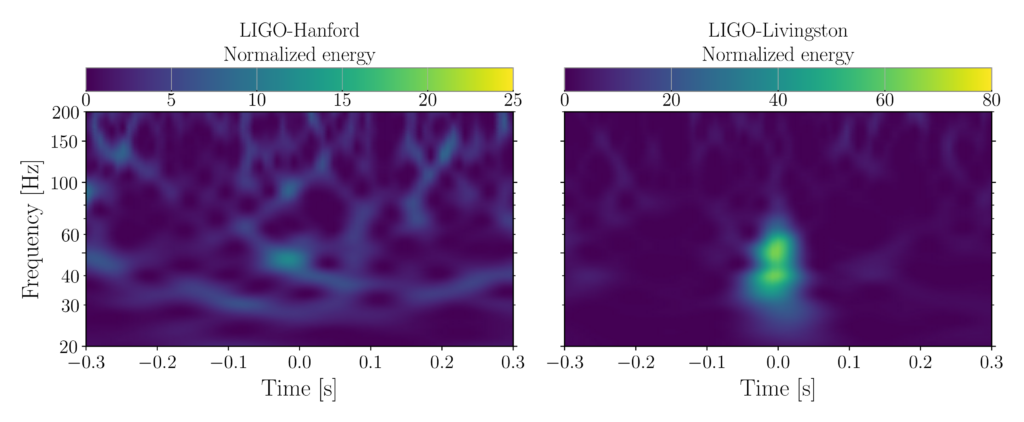
Figure 3: (Figure 5 from the paper) Spectrograms of the most significant new candidate identified by the eBBH search for the LIGO-Hanford (left) and LIGO-Livingston (right) detectors. The individual detector signal-to-noise ratios in the LIGO-Hanford and LIGO-Livingston are 5.6 and 10.9 respectively. Since the energies in the two detectors are very different, we use different scales on the colorbar.
What our results imply
Although our search did not identify a high significance eBBH candidate, we used this non-detection to determine astrophysical implications of our results by characterizing our search’s sensitivity to such systems. To do this, we injected simulated numerical relativity signals with a range of total masses (from 70 M☉ to 200 M☉) and mass ratios (q from 0.33 to 1.0) into data from the third observing run of LIGO and Virgo, and recovered them using the eBBH search. Using these results, we were able to quantify the sensitive volume-time of our search as a function of eBBH source parameters (see Figure 4). The sensitive volume-time we calculated for our search sets an upper limit on the merger rate of binary black holes in AGNs and dense star clusters which is consistent with what population-modelers predict.
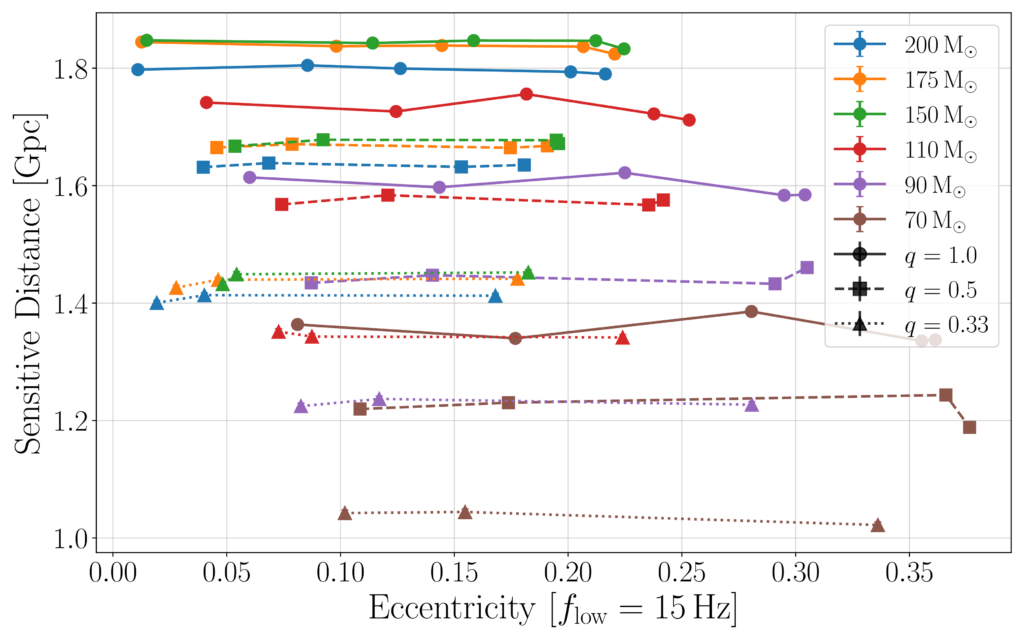
Figure 4: (Figure 4 from the paper) This figure demonstrates how far we can see eBBH systems (in gigaparsecs, where 1 gigaparsec is equivalent to 3.26 billion light-years) as a function of orbital eccentricity for different source total masses and mass ratios. The marker shapes represent systems with different mass ratios and the colors represent the various total masses (in units of the Sun’s masses) considered here. The horizontal axis denotes the eccentricity of the binary at an orbital separation that corresponds to an emission frequency of 15 Hz.
Find out more:
Back to the overview of science summaries.