What is dark matter? Scientists have been asking themselves this question for nearly a century, but it remains a big mystery in the universe. Astrophysical observations have revealed that something invisible but heavy – dark matter – plays a critical role in forming galaxies and stars by pulling ordinary visible matter together with gravity. We now know that dark matter accounts for 85% of all the matter in our universe, but we still don’t know what are its constituents. For example, dark matter could consist of primordial black holes, each weighing up to hundreds of solar masses, or it could be a new particle with a mass similar to our known heaviest elementary particles, or it could be an ultralight boson particle lighter than electrons by tens of orders of magnitude. So far, all the past experiments and observations have failed to make a convincing detection of any such dark matter candidates.
LIGO, Virgo and KAGRA are gravitational-wave detectors which measure tiny length changes caused by gravitational waves passing through their laser interferometers. Although they are designed primarily for gravitational-wave detection, they are also sensitive to certain types of dark matter which also cause length changes. One specific dark matter candidate is called vector boson dark matter. Just like charged masses in an electromagnetic field feel electromagnetic forces, masses in a vector field that have a different kind of “charge“ beyond the standard model of particle physics feel non-standard forces. Ultralight vector dark matter creates oscillatory forces on any objects carrying such charges. These can be mirrors in a laser interferometer. The “charge“ can be the baryon number, which simply counts the number of protons and neutrons in a mirror, or the baryon minus lepton number, or B-L, which equals the number of neutrons. Laser interometers measure the oscillatory length changes between mirrors, caused by the forces from vector dark matter. In 2021, the LIGO–Virgo–KAGRA collaboration used the data from the third observing run (O3) of Advanced LIGO and Advanced Virgo and placed the best upper limits on the strength of such forces from vector dark matter.
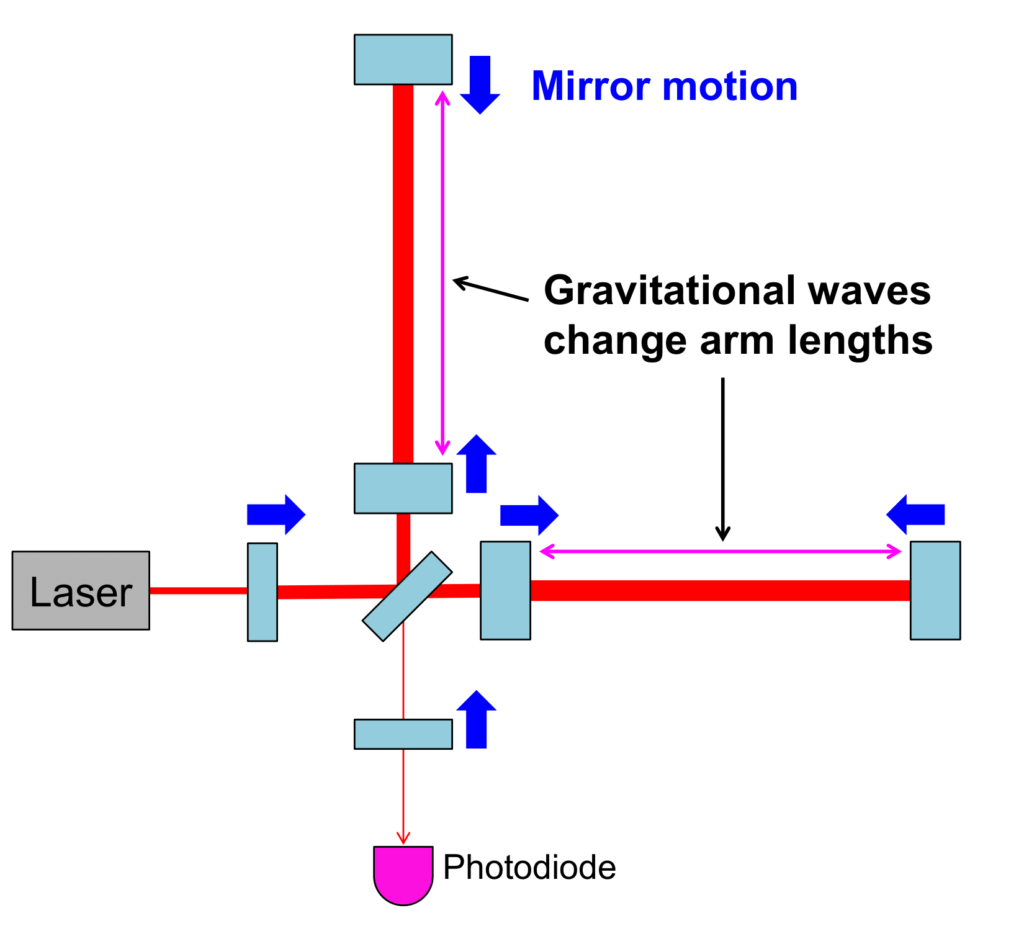
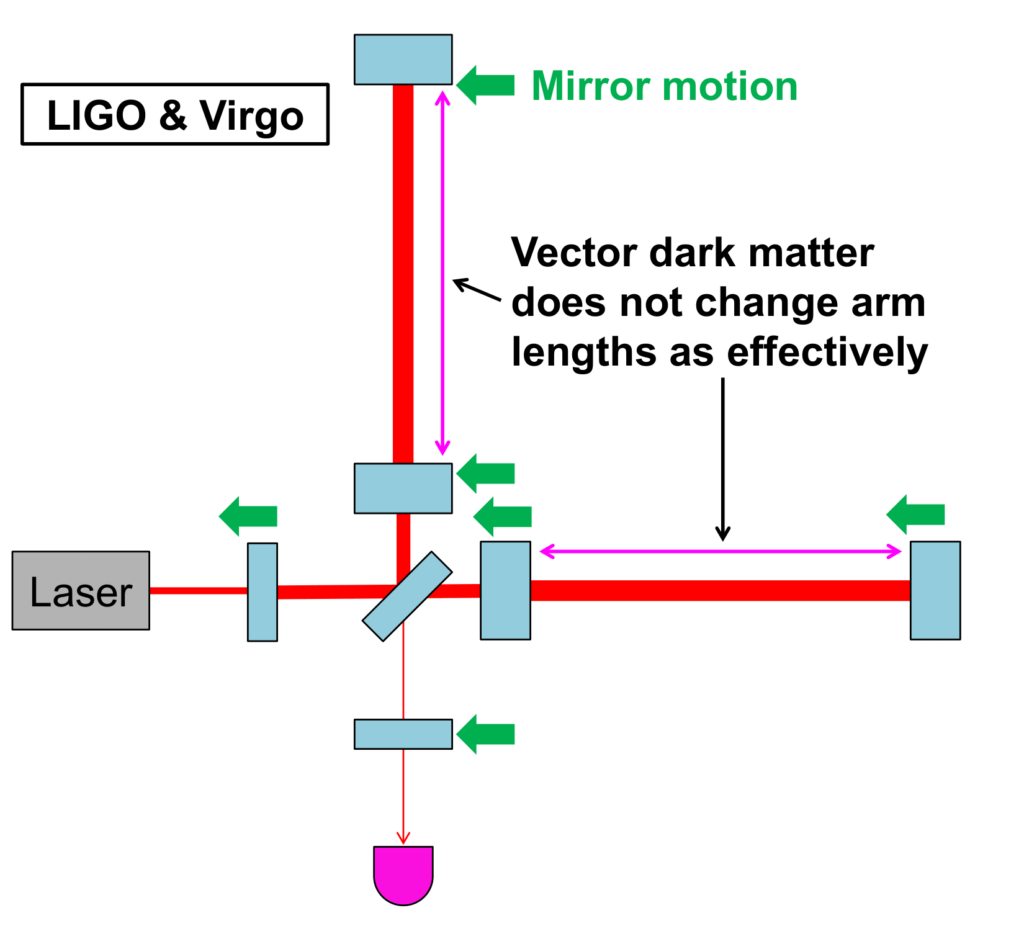
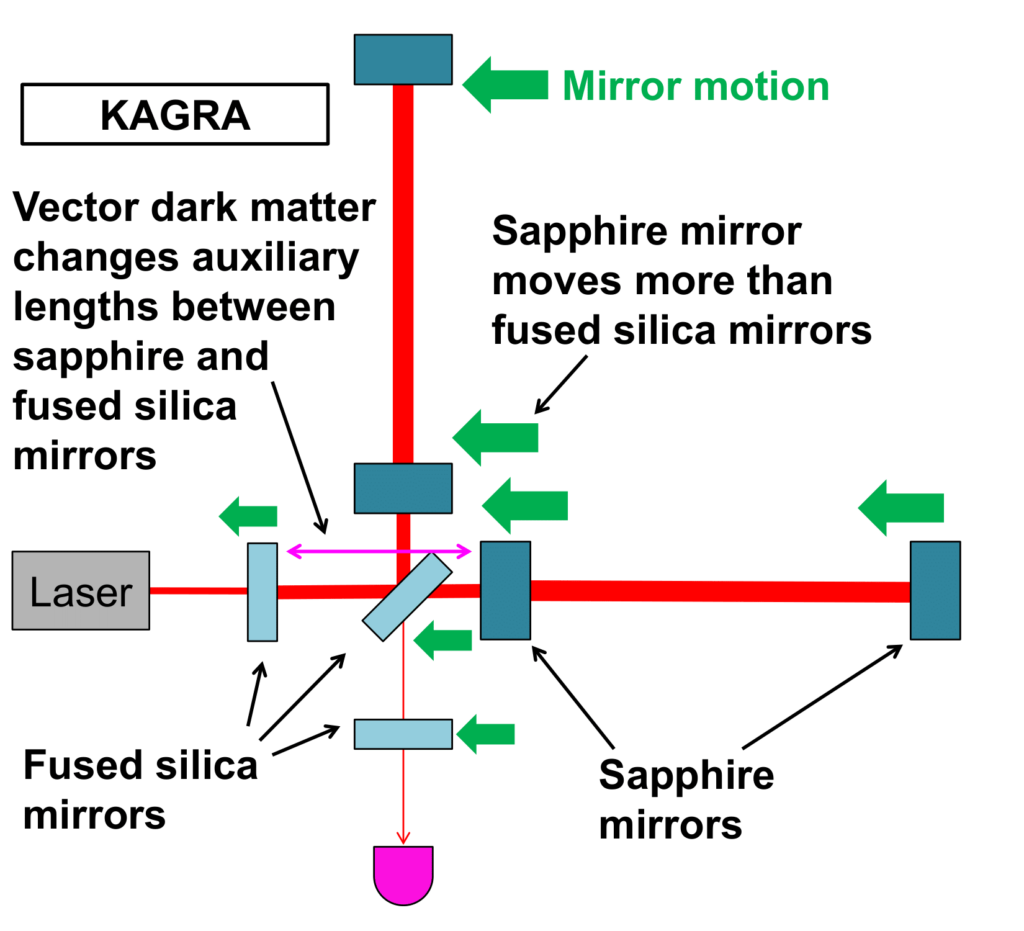
Figure 1: Infographics of the laser interferometer response to gravitational waves (top), to vector dark matter in LIGO and Virgo case (middle), and to vector dark matter in KAGRA’s auxiliary lengths, which records the distance between sapphire and fused silica mirrors (bottom).
In LIGO and Virgo, all the mirrors are made from fused silica glass, and all the mirrors move almost perfectly in common in the vector dark matter field because the charge-to-mass ratio is the same between all the mirrors. Laser interferometers are sensitive to the distance changes between mirrors, but not very sensitive when the mirrors move in common and the distance stays unchanged. In LIGO and Virgo’s case, the sensitivity to vector dark matter is about five orders of magnitude less than when the mirrors move differentially. The KAGRA detector in Japan is unique in using sapphire for four main test mass mirrors and fused silica for other auxiliary mirrors. For dark matter coupled to the B-L number, sapphire mirrors move slightly more than fused silica mirrors because they are slightly more neutron-rich. Therefore, by measuring the distance between sapphire and fused silica mirrors, KAGRA can be more sensitive to vector dark matter than LIGO and Virgo, especially for lighter dark matter particles.
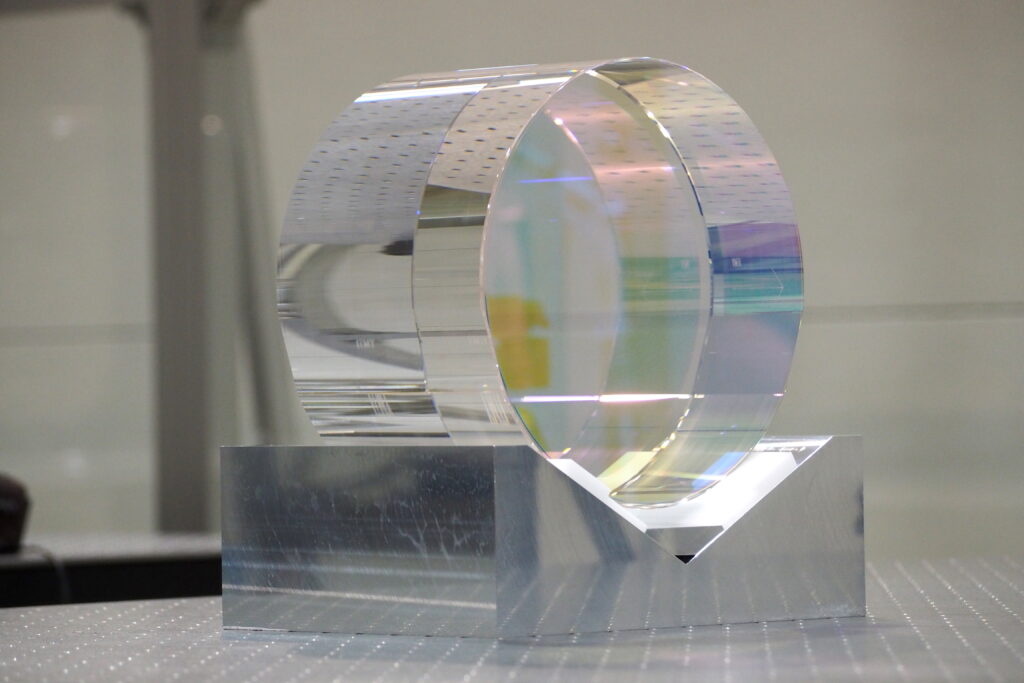
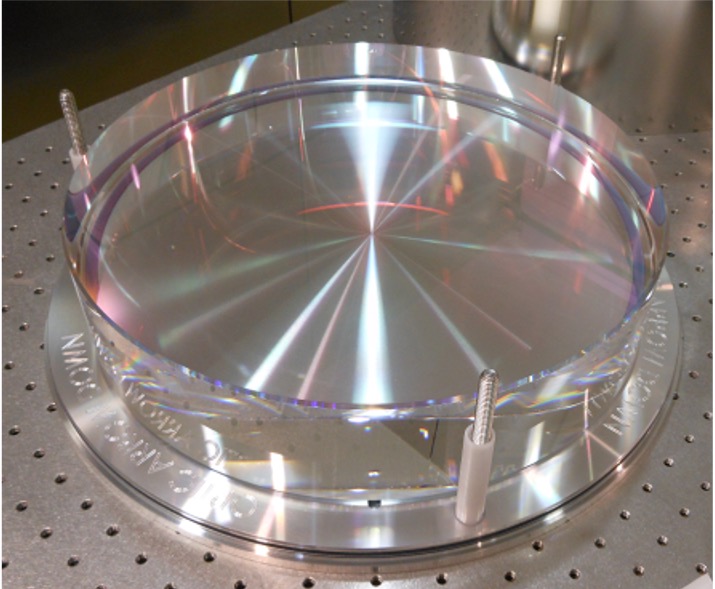
Figure 2: A sapphire mirror (top) and a fused silica mirror (bottom) were used for the KAGRA interferometer. Although they both look transparent and similar to our eyes, the sapphire mirror is slightly more neutron-rich, moving more than fused silica mirrors when interacting with B-L vector dark matter.
In this study, we used the data from KAGRA’s first joint observing run together with the GEO600 detector in Germany (O3GK run). We have developed a new pipeline to search for oscillatory length changes. Since the mass of ultralight vector dark matter determines the frequency of the oscillatory length changes, we can search for dark matter particles at different masses by searching for length changes at different frequencies. Because of the statistics of ultralight dark matter particles, the strength of such length changes fluctuates at a timescale called coherent time. Therefore, we carefully accounted for the stochastic nature of the signals from ultralight dark matter in our search. During O3GK, KAGRA took the data from auxiliary length channels which record the distance changes between sapphire test masses and fused silica auxiliary mirrors. By analyzing more than 100 hours of data, we found no evidence of dark matter signals and therefore set upper limits on how strong the forces from the B-L vector dark matter could be.
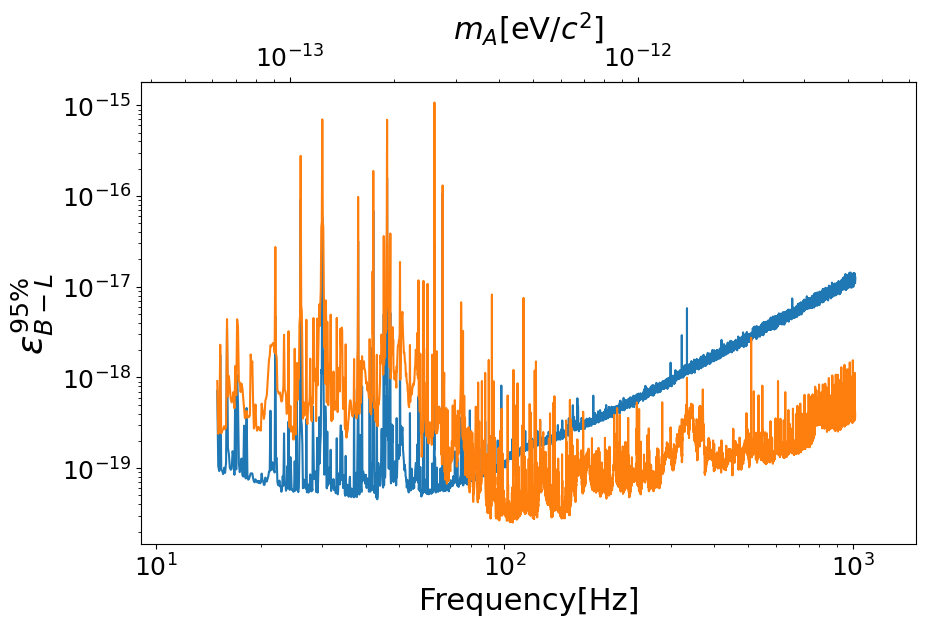
Figure 3: Upper limits on the strength of forces from B-L vector dark matter from KAGRA data. The two curves are from different auxiliary length channels. The strength is expressed in terms of a fraction of the electromagnetic force. For more information on this figure and how it was produced, read the freely available preprint.
Since KAGRA had not yet reached its planned sensitivity at the time of the O3GK run, our limits are orders of magnitude less stringent than those from previous experiments. Still, our study has demonstrated the feasibility of using auxiliary length channels of gravitational-wave detectors like KAGRA for astrophysical observations. When the O3GK run was conducted in 2020, auxiliary length channels were not even considered useful for observational science. The main gravitational-wave channel records the distance changes between sapphire mirrors, and the auxiliary length channels are usually used only to keep this main channel sensitive to gravitational waves. With dedicated noise reductions in auxiliary length channels in future runs, KAGRA can give us insight into the question: what is dark matter?
Find out more:
Back to the overview of science summaries.