In August 2017 Advanced LIGO and Advanced Virgo detected gravitational waves from a binary neutron star merger for the very first time. This ground-breaking event was also observed extensively by telescopes sensitive to light across the entire electromagnetic spectrum, allowing the host galaxy of the gravitational-wave source to be clearly identified. By combining the galaxy distance measured from the gravitational-wave data with its radial velocity measured from electromagnetic data, we have made a novel and entirely independent determination of an important quantity in cosmology: the present-day expansion rate of the universe. This measurement marks the dawn of an exciting, new era of gravitational-wave cosmology.
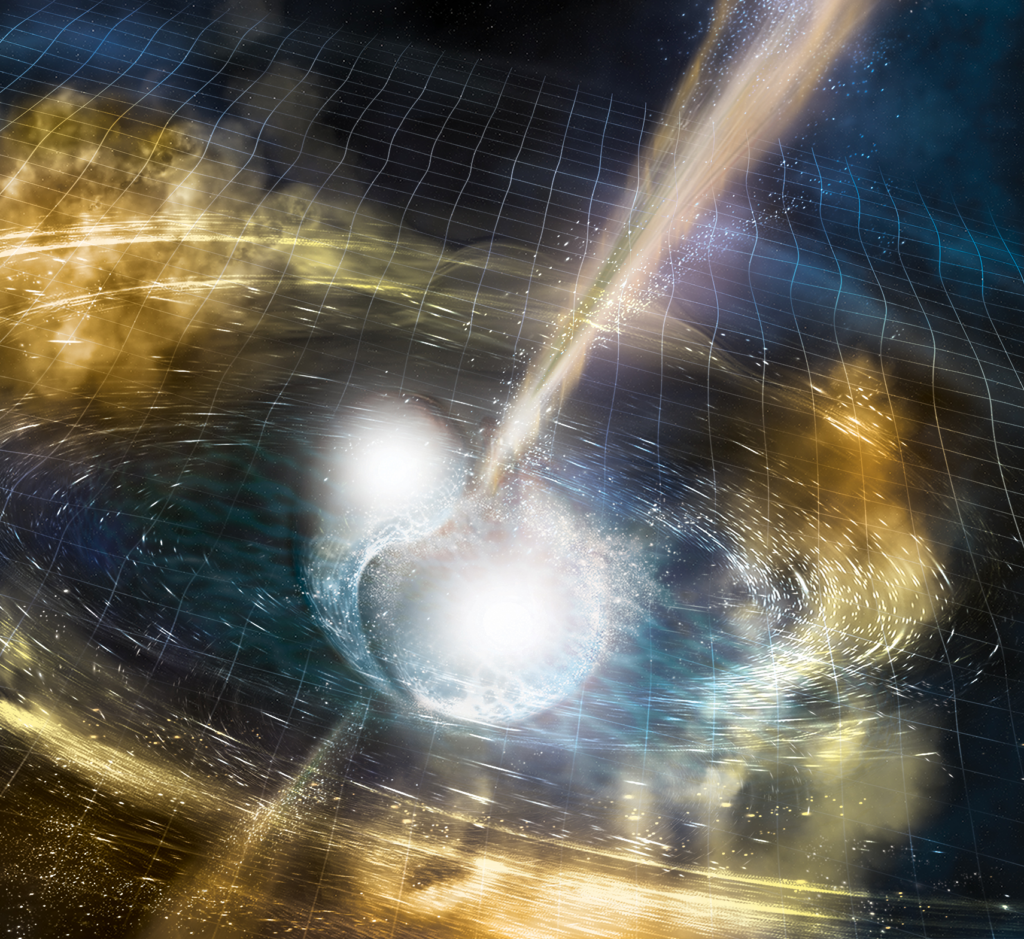
Figure 1: Artist’s illustration of two merging neutron stars. The narrow beams represent the gamma-ray burst while the rippling spacetime grid indicates the isotropic gravitational waves that characterize the merger. Swirling clouds of material ejected from the merging stars are a possible source of the light that was seen at lower energies. Credit: National Science Foundation/LIGO/Sonoma State University/A. Simonnet.
A Brief History of the Hubble Expansion
It is nearly 90 years since astronomers first learned that our universe is expanding – a ground-breaking discovery that revolutionised our understanding of the cosmos, underpinning the Big Bang Theory for its origin and evolution, and now represents one of the cornerstones of modern cosmology.
At the vanguard of that discovery was Edwin Hubble, after whom the Hubble Space Telescope is named; his observations of galaxies in the nearby universe revealed a linear relationship between their distance and their recession velocity away from the Milky Way (see Figure 2) consistent with the interpretation that the galaxies were generally not moving through space but were being carried apart by the expansion of space itself. This phenomenon of universal expansion now bears Hubble’s name and the current rate of expansion, equal to the constant of proportionality in Hubble’s linear relationship, is known as the Hubble constant. It is denoted by the symbol H0 and is expressed in units of kilometres per second per megaparsec (denoted Mpc: one parsec is a unit of distance equal to about three and a quarter light years or 3.086 x 1016 metres). Hubble’s original estimate for H0 was about 500 kms-1 Mpc-1 (see Figure 2) but this value was later realised to be a very significant over-estimate.
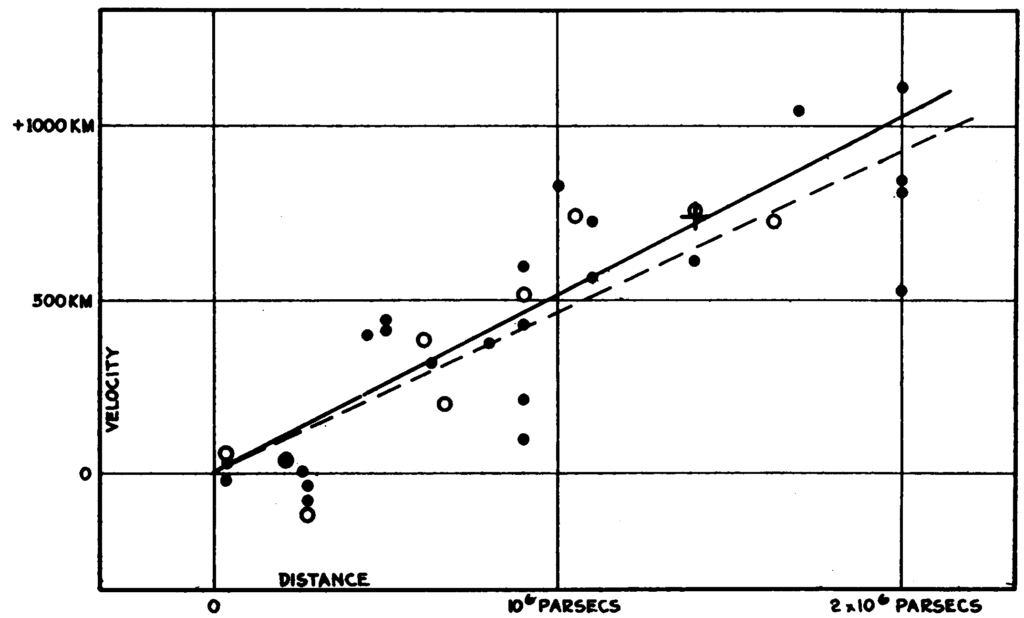
Figure 2: Schematic plot of the Hubble relation, adapted from Edwin Hubble’s original 1929 data. The linear relationship between radial velocity (vertical axis, wrongly labelled in kilometres in Hubble’s original paper) and distance (horizontal axis, labelled in parsecs) is clearly apparent – although there is considerable scatter in the data and the distance range extends to only about 2 Mpc. Credit: Proc. Nat. Acad. Sciences. (1929)
By the early 21st century a wide range of different methods to measure the Hubble constant had been developed and applied, drawing upon a variety of different types of astrophysical data – including observations of Cepheid variable stars, type Ia supernovae, the Tully-Fisher relation and even the cosmic microwave background (CMB). A key feature of many H0 measurements that use data from the nearby universe is the requirement to combine together two or more distance indicators – employing what is often referred to as the Cosmic Distance Ladder. Clearly methods that are able to bypass the “rungs” of this ladder could be very useful, and we describe one such completely novel approach in this paper.
Of the current ‘state-of-the-art’ measurements of the Hubble constant we highlight here two contrasting methods that are applied on nearby and very distant cosmological scales respectively, and are currently inconsistent with each other: the ShoES project, which combines Cepheids and type Ia supernovae, gives a Hubble constant that is about 8% larger than the value derived from CMB observations by the Planck satellite. While this discrepancy might not seem too large, and is much smaller than the huge inconsistencies between different Hubble constant estimates that plagued much of the 20th century, it nevertheless represents a significant, unresolved puzzle for cosmology.
A New Approach: Standard Sirens
We present here a novel approach to measuring the Hubble constant using gravitational waves, applying for the very first time a revolutionary method proposed more than 30 years ago in a seminal paper by Bernard Schutz and later refined in this article. The key idea of this method is to estimate the distance of a galaxy using gravitational-wave observations of a so-called “standard siren“, which is the gravitational analogue of an astronomical “standard candle” – the luminosity (and hence distance) of which can be inferred directly from observations. Standard sirens are compact binary systems, consisting of neutron stars or black holes, whose evolving “chirp“-like gravitational waveform as the compact objects inspiral towards merger carries information about the distance of the source – as well as the masses of the compact objects and other parameters of the system. By analysing the waveform, we can estimate that distance. We have used the gravitational-wave source GW170817, which was detected by Advanced LIGO and Advanced Virgo on August 17 2017, to make the first-ever standard siren measurement of the Hubble constant.
GW170817 represents the very first time that both gravitational and electromagnetic waves from a single astrophysical source have been observed. This joint observation supports the hypothesis that the source is the merger of two neutron stars and it also allowed for the identification of the host galaxy – a galaxy known as NGC4993 in the constellation of Hydra. Analysis of the waveform of GW170817 yielded a distance estimate of about 44 Mpc assuming that the sky position of GW170817 was exactly coincident with its optical counterpart. This distance estimate carried an uncertainty of about 15%, resulting from a combination of instrumental noise in the detectors and the fact that we don’t know precisely the inclination of the orbital plane of the binary neutron star system with respect to the Earth. (See also Figure 4). Nevertheless, our gravitational-wave distance estimate has the great virtue of being completely independent of the Cosmic Distance Ladder derived from electromagnetic observations.
A Standard Siren Measurement of H0
To estimate H0 we need to combine our gravitational-wave distance to NGC4993 with the galaxy’s radial velocity due to the Hubble expansion. We can’t simply use the measured radial velocity of NGC4993, however. This is because the expansion of the universe is not smooth and uniform everywhere: the ‘clumpiness’ of the universe distorts the Hubble expansion on smaller scales because galaxies have additional motions (referred to as “peculiar velocities“) due to the gravitational attraction of other galaxies and clusters (and unseen dark matter too) in their vicinity. The relative closeness of NGC4993 means that its peculiar velocity is likely to be a significant part of its measured radial velocity and can’t be ignored.
We accounted for the peculiar velocity of NGC4993 by first identifying the small group of galaxies with which it is associated, and then used results from a much larger survey of galaxy peculiar velocities to correct for the motion of that group as a whole. This gave a “Hubble velocity” estimate for NGC4993 (i.e. the velocity that it would have if it were simply being carried along by the Hubble expansion) of just over 3000 kms-1. We checked this result by comparing it with other methods for reconstructing the pattern of galaxy peculiar velocities on large scales, and found them to be fully consistent with each other. As a precaution we allowed for a large uncertainty of 150 kms-1 in our peculiar velocity correction, while recognising that the dominant effect on our estimation of H0 is still the 15% uncertainty on our gravitational-wave distance.
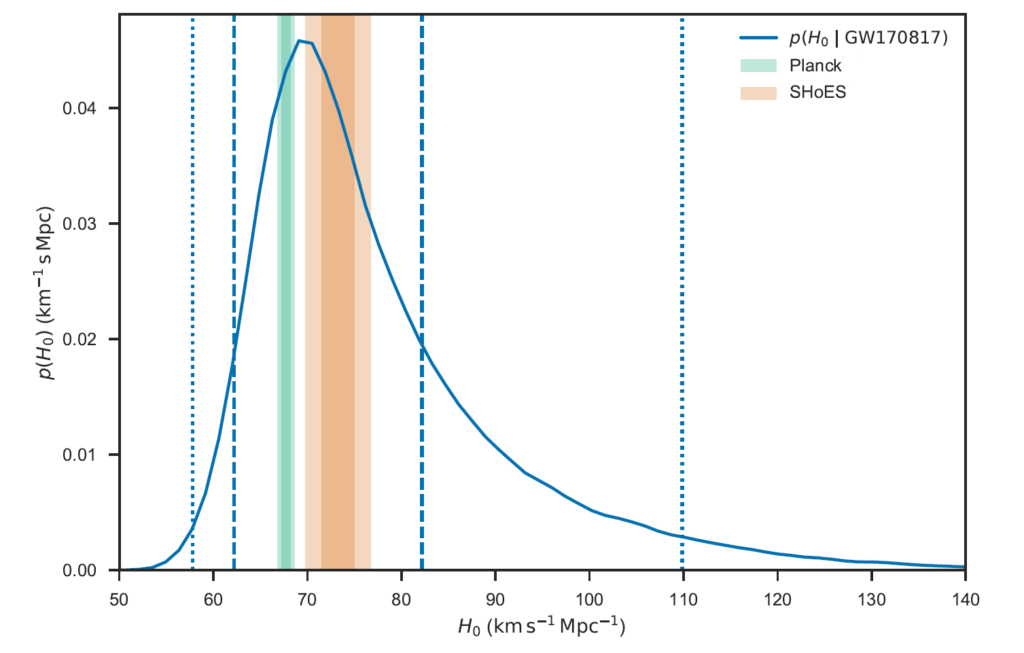
Figure 3: Plot summarising our inference on the Hubble constant. The relative probability of different values of H0 is represented by the solid blue curve, which peaks at 70 kms-1 Mpc-1. The dashed and dotted blue vertical lines respectively show the limits of 68.3% and 95.4% credible intervals for H0. The vertical green and orange bands represent the constraints on H0 from two state-of-the-art analyses using solely electromagnetic data: the green bands show the range of values inferred from analysis of CMBR data obtained by the Planck satellite; the orange bands show the range of values inferred from the SHoES analysis that combines Cepheid variable and type Ia supernovae data from the relatively nearby universe. The darker and lighter coloured bands indicate 68.3% and 95.4% credible intervals respectively. Note that the Planck and SHoES results are not in agreement with each other at the 95.4% probability level. Our gravitational-wave result is, however, consistent with both the Planck and SHoES values.
Figure 3 summarises our measurement of the Hubble constant. The solid blue curve represents the probability of different values of H0 with the peak at 70 kms-1 Mpc-1, and the dashed and dotted lines respectively mark out the limits of different credible intervals for H0 – i.e. the ranges within which our analysis indicates that the Hubble constant lies with a given probability. Figure 4 then shows the joint constraints on H0 and the inclination of the binary neutron star system and illustrates that there is a degeneracy between these quantities, meaning that our data assigns similar probabilities to certain combinations of H0 and inclination. This degeneracy arises because of the significant correlation between distance and inclination angle for a gravitational-wave source: the gravitational-wave amplitude emitted by a distant binary viewed face-on or face-off is similar to that of a closer binary viewed edge-on. It also causes the extended low-probability ‘tail’ for high values of H0 in Figure 3, since the solid blue curve in that figure is essentially obtained by projecting along the vertical axis the contour plot in Figure 4.
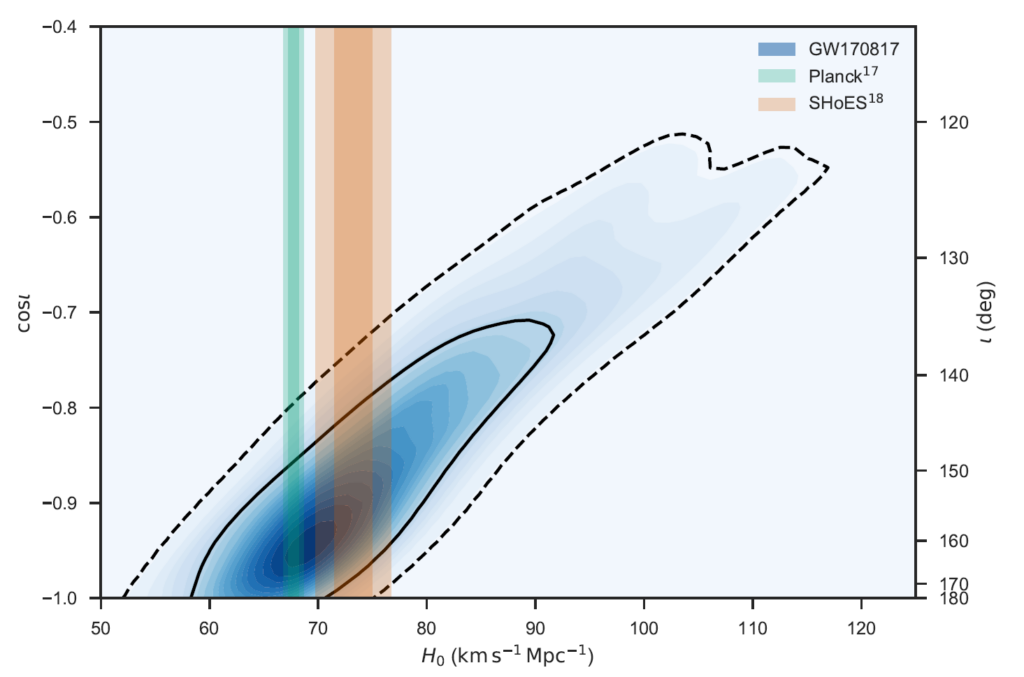
Figure 4: Contour plot summarising what our analysis infers jointly about the value of the Hubble constant (horizontal axis) and the inclination of the binary neutron star system’s orbital plane (vertical axis; the right-hand axis shows inclination angle in degrees and the left-hand axis shows cosine of the inclination angle). The darkest shading shows the region of highest probability. Note the significant correlation between inclination angle and inferred Hubble constant. This is because of the significant correlation between distance and inclination angle for a gravitational-wave source: the gravitational-wave amplitude emitted by a distant binary viewed face-on or face-off is similar to that of a closer binary viewed edge-on. The highest probability region corresponds to inclination angles close to 180 degrees – indicating that GW170817 was viewed almost face-off.
Conclusions
We have carried out the first ever measurement of the Hubble constant using gravitational-wave and electromagnetic observations. Our estimate is consistent with the values of H0 found using both nearby and very distant cosmological data, represented by the SHoES and Planck analyses respectively, but crucially it has been derived completely independently of the traditional Cosmic Distance Ladder. This marks an important milestone in the long history of measuring the expansion rate of the universe, and we anticipate that future gravitational-wave observations will be able to make increasingly precise measurements of this fundamental quantity. The era of gravitational-wave cosmology has truly arrived!
Find out more:
- Visit our websites: http://ligo.org, http://www.virgo-gw.eu
- Visit the LIGO Open Science Center, with access to GW170817 data: https://losc.ligo.org
- Multi-messenger Observations of a Binary Neutron Star Merger
- GW170817: Observation of gravitational waves from a binary neutron star inspiral
- Gravitational Waves and Gamma Rays from a Binary Neutron Star Merger: GW170817 and GRB 170817A
- A gravitational-wave standard siren measurement of the Hubble constant with GW170817
- Search for post-merger gravitational waves from the remnant of the binary neutron star merger GW170817
- On the Progenitor of Binary Neutron Star Merger GW170817
- GW170817: Implications for the Stochastic Gravitational-Wave Background from Compact Binary Mergers
- Search for High-energy Neutrinos from Binary Neutron Star Merger GW170817 with ANTARES, IceCube, and the Pierre Auger Observatory