On August 17, 2017 astronomers around the world were alerted to gravitational waves observed by the Advanced LIGO and Advanced Virgo detectors. This gravitational wave event, now known as GW170817, appeared to be the result of the merger of two neutron stars. Less than two seconds after the GW170817 signal, NASA’s Fermi satellite observed a gamma-ray burst, now known as GRB170817A, and within minutes of these initial detections telescopes around the world began an extensive observing campaign. The Swope telescope in Chile was the first to report a bright optical source (SSS17a) in the galaxy NGC 4993 and several other teams independently detected the same transient over the next minutes and hours. For the next several weeks astronomers observed this location with instruments sensitive across the electromagnetic spectrum; these observations provide a comprehensive view of this cataclysmic event starting ~100 seconds before merger until several weeks after. The observations support the hypothesis that two neutron stars merged in NGC 4993 – producing gravitational waves, a short-duration gamma-ray burst, and a kilonova. GW170817 marks a new era of multi-messenger astronomy, where the same event is observed by both gravitational waves and electromagnetic waves.
Introduction
The idea of a neutron star (NS) was first presented over eighty years ago in 1934, but it was another 33 years before they were observed. In 1967 X-ray emission from Scorpius X-1 was determined to be from a NS, and later the same year the first radio pulsar was discovered. Since then several binary neutron star (BNS) systems have been discovered, including the Hulse-Taylor binary, a BNS where one of the NSs is a pulsar. BNS have provided strong observational tests of General Relativity including the first firm evidence for the existence of gravitational waves (GWs). Since the early days of LIGO, BNS mergers have been considered a primary target for gravitational wave observations.
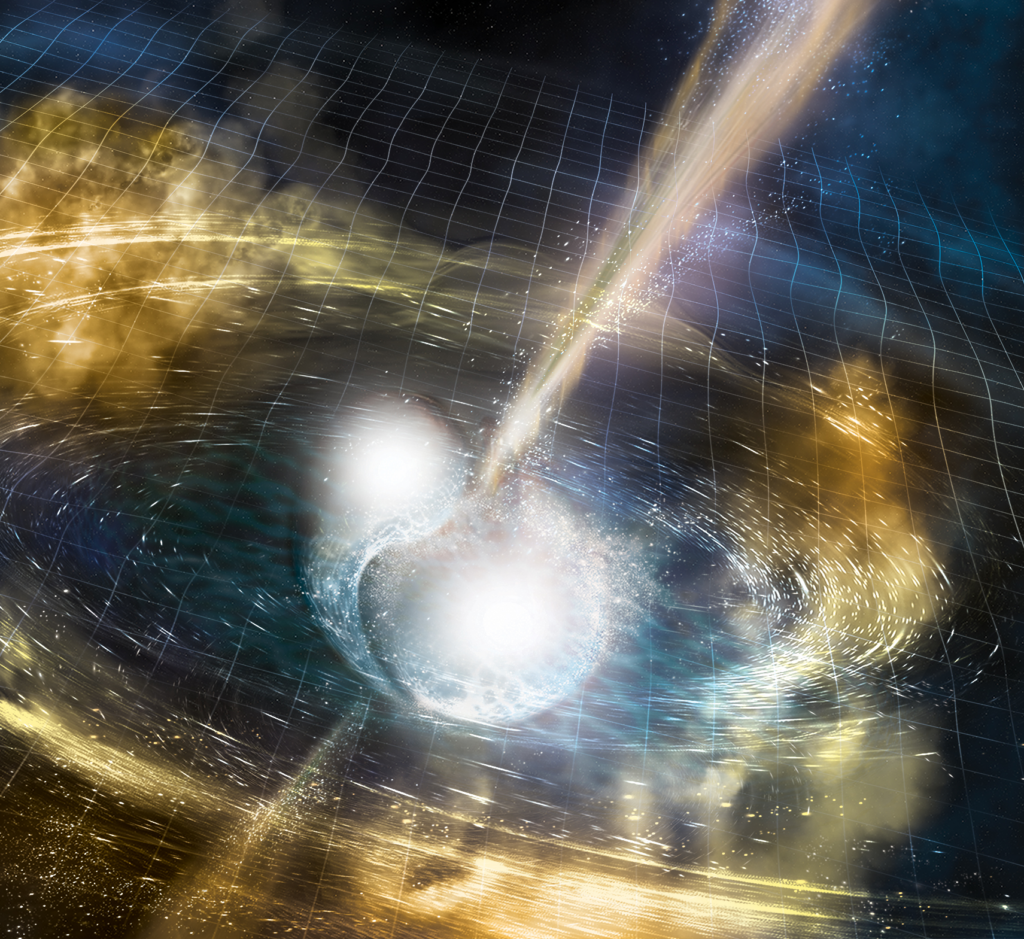
Artist’s illustration of two merging neutron stars. The narrow beams represent the gamma-ray burst while the rippling spacetime grid indicates the isotropic gravitational waves that characterize the merger. Swirling clouds of material ejected from the merging stars are a possible source of the light that was seen at lower energies. Credit: National Science Foundation/LIGO/Sonoma State University/A. Simonnet.
In the mid-1960s gamma-ray bursts (GRBs) were discovered by the Vela satellites, and later established to be of cosmic origin. Determining the sources of GRBs has been one of the key challenges in high-energy astrophysics ever since. The idea that GRBs might be related to BNS mergers had been put forward early on and in 2005 the field experienced a breakthrough, when a short-duration gamma-ray burst (sGRB) was localized to a host galaxy, and multi-wavelength (X-ray, optical, radio) afterglows could be observed. These multi-wavelength observations provided evidence that sGRBs might be associated with BNS mergers or the merger of a NS with a black hole.
A Multi-Messenger Discovery
On August 17, 2017 NASA’s Fermi satellite and its Gamma-ray Burst Monitor (GBM) instrument sent an automatic alert about GRB170817A. It took about 6 minutes for automated LIGO data analysis to find that a candidate GW transient (later designated GW170817) had been detected at almost the same time at the LIGO-Hanford observatory. The GW was consistent with a BNS merger occurring less than 2 seconds before GRB170817A and the LIGO-Virgo rapid-response team manually inspected the data and issued an alert, reporting that a highly significant GW candidate was associated with the time of the GRB. Initial analysis of the data identified the area of the sky most likely to be the source of the GRB170817A and GW170817 signals, shown in Figure 1.
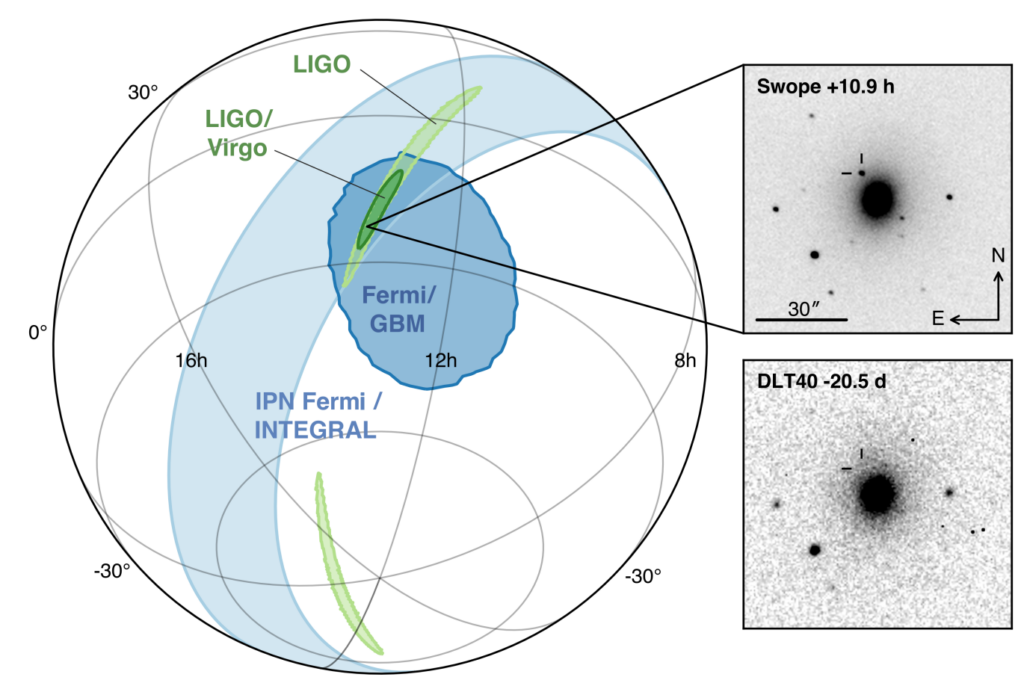
Figure 1: Localization of the gravitational-wave, gamma-ray, and optical signals. The left panel shows a projection of the 90% credible regions from LIGO (light green), LIGO-Virgo (dark green), triangulation from the time delay between Fermi and INTEGRAL (light blue), and Fermi GBM (dark blue). The inset shows the location of the apparent host galaxy NGC 4993 in the Swope optical discovery image at 10.9 hours after the merger (top right) and the DLT40 pre-discovery image from 20.5 days prior to merger (bottom right).
This event marked the first GW multi-messenger discovery: it was observed by both GWs and electromagnetic (EM) waves. With the area of the sky identified from the GW and gamma-ray signal, telescopes around the world focused their effort to make further observations associated with this source. There was a plethora of key observations that occurred at different electromagnetic wavelengths, as well as neutrino fluence measurements, and Figure 2 shows a timeline of the observations. The multi-wavelength observations were critical to the richness of this scientific discovery.
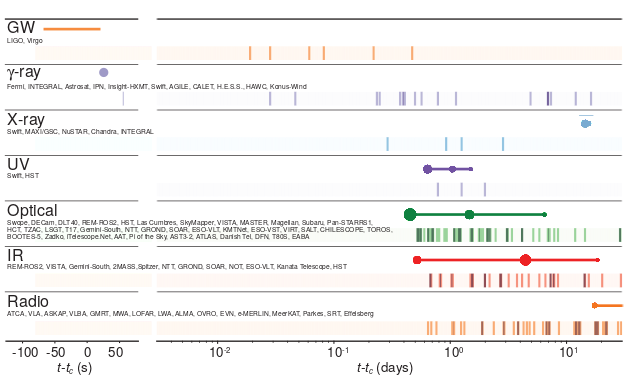
Figure 2: The timeline for the discovery of GW170817, GRB170817A, SSS17a/AT2017gfo and the follow-up observations are shown in Figure 2 from the publication. Some of the elements of that figure are presented here and described in more detail. Figure 2a: this shows the timeline of the discovery and follow-up of the system. Two types of information are shown for each band/messenger. First, the shaded dashes represent the times when information was reported in a GCN Circular. The names of their associated authors is collected at the beginning of the row. Second, the solid lines with circles indicate when the source was detectable by at least one telescope in the particular band. The circle sizes are scaled approximately by magnitude.
At the time of the alert for GW170817, the location of the source in the sky had set in Australia, but it was still well placed for observing by telescopes in South Africa and Chile. In the first few hours of Chilean darkness, the Swope telescope identified an optical transient (SSS17a) in the galaxy NGC 4993. Over the next two weeks, a network of ground-based telescopes and space-based observatories followed up the initial detections, spanning the ultraviolet (UV), optical (O), and near infrared (IR) wavelengths. These observations carefully monitored the spectral energy distribution, revealing that this exceptional electromagnetic counterpart was a kilonova. This observation firmly connects kilonovae with the BNS merger, providing evidence supporting the idea that kilonovae result from the radioactive decay of the heavy elements formed by neutron capture during a BNS merger.
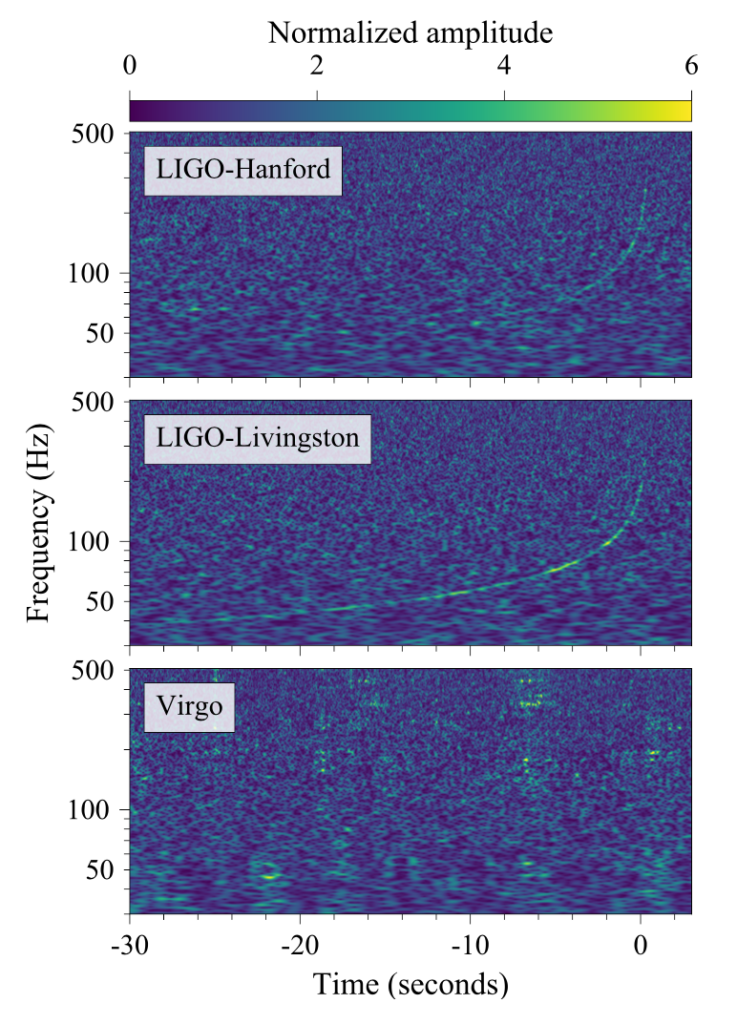
Figure 2b: shown here is a spectrogram of the gravitational waves as seen in the LIGO-Livingston detector. Here we show the spectrograms from all three LIGO-Virgo detectors. You can see the characteristic “chirp”, when the frequency increases, of a binary merger.
Following the kilonova, X-ray and radio observatories studied the source, recording a counterpart signal from the event. These observations revealed important information on the energy output of the explosion, the ejected material, and the environment of the merger. Neutrino observatories searched for coincident, high-energy neutrinos from the area of GW170817 as neutrinos are emitted in the relativistic outflow produced during a BNS merger. However, no neutrinos were identified that came from the direction of GW170817 and no supernova neutrino burst signal was detected coincident with the merger. Following the identification of the host galaxy of the event, an extended search for neutrinos in the direction of NGC 4993 was carried out for two weeks following the merger, but it found no significant neutrino emission. It remains a goal of multi-messenger astronomy to detect gravitational waves, electromagnetic radiation, and neutrinos from the same cosmic event.
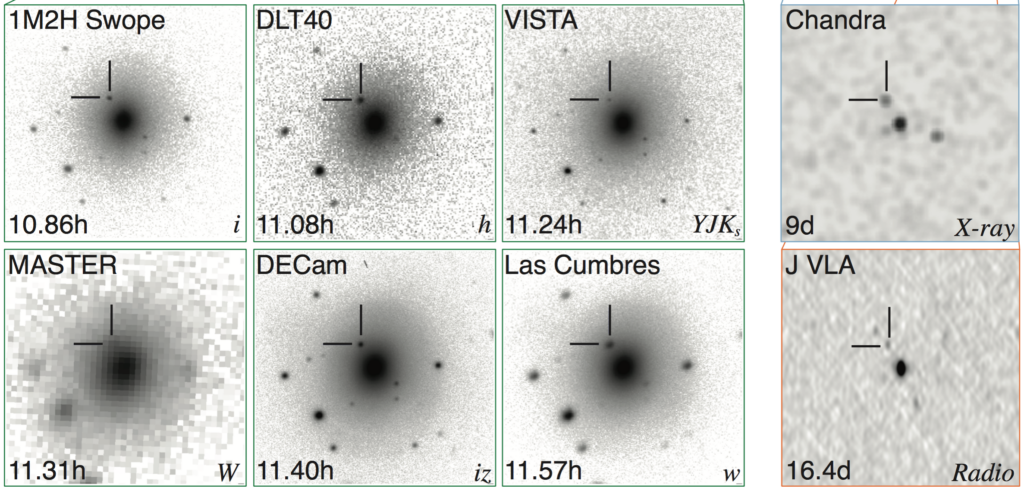
Figure 2c: shown here are 8 images of the aftermath of the BNS merger (designated SSS17a/AT2017gfo). On the left are six optical images taken between 10 and 12 hours after the merger by different telescopes. On the right are images constructed from x-ray and radio observations. The x-ray image was taken 9 days after the merger by NASA’s Chandra X-ray Observatory. 16 days after the merger NRAO’s Jansky Very Large Array (VLA) captured the radio image. In all 8 images the galaxy NGC 4993 is seen in the middle and SSS17a/AT2017gfo is marked by two lines.
Conclusions
For the first time, both gravitational and electromagnetic waves from a single astrophysical source have been observed. This joint observation supports the hypothesis that the source is the merger of two neutron stars and it also allowed for the identification of the host galaxy. The electromagnetic observations comprise three major components at different wavelengths: (i) a prompt, short gamma-ray burst which demonstrates the association of at least a fraction of these phenomena with mergers of neutron star binaries, (ii) an ultraviolet, optical, and infrared transient (known as a kilonova), due to the radioactive decay of heavy elements formed by neutron capture, observed clearly for the first time, followed by (iii) delayed X-ray and radio counterparts. All of these observations provide the first global picture of the processes at play after compact star mergers that contain neutron stars, including a jet of high-energy particles and the interaction of this jet with the surrounding interstellar medium. This event also demonstrates the importance of collaborative, joint gravitational-wave, electromagnetic, and neutrino observations, and marks a new era in multi-messenger, time-domain astronomy.
Find out more:
- Visit our websites: http://ligo.org, http://www.virgo-gw.eu
- You can read the full article, which is published in Astrophysical Journal Letters, here.
- Visit the LIGO Open Science Center, with access to GW170817 data: https://losc.ligo.org
- GW170817: Observation of gravitational waves from a binary neutron star inspiral
- Gravitational Waves and Gamma Rays from a Binary Neutron Star Merger: GW170817 and GRB 170817A
- A gravitational-wave standard siren measurement of the Hubble constant with GW170817
- Search for post-merger gravitational waves from the remnant of the binary neutron star merger GW170817
- Estimating the Contribution of Dynamical Ejecta in the Kilonova Associated with GW170817
- On the Progenitor of Binary Neutron Star Merger GW170817
- GW170817: Implications for the Stochastic Gravitational-Wave Background from Compact Binary Mergers
- Search for High-energy Neutrinos from Binary Neutron Star Merger GW170817 with ANTARES, IceCube, and the Pierre Auger Observatory