In 2016 the first direct detections of gravitational-wave signals were announced by the LIGO Scientific Collaboration and Virgo Collaboration. So far, the signals detected by the Advanced LIGO and Advanced Virgo detectors were all due to transient signals emitted by the collision and merger of compact binary systems comprising pairs of black holes or neutron stars. However, there are other astrophysical sources expected to emit detectable gravitational-wave signals. Among those are isolated rapidly rotating neutron stars — compact remnants of supernova explosions with roughly 1.5 times the mass of our Sun and a diameter of only about 20 kilometers.
If these compact stellar remnants are not perfectly round, e.g. if they have a “mountain” (most likely not more than a meter-high bump) on their surface, they are expected to emit persistent (or continuous) gravitational waves. How much the neutron star’s shape differs from a perfect sphere is measured by a quantity called the “ellipticity.” The motion of the gravitational-wave detector due to the Earth’s rotation and its revolution around the Sun causes a frequency shift of the nearly-sinusoidal (continuous) signal emitted by the rapidly rotating neutron star. This is due to the Doppler effect, which will shift the received frequency of the gravitational waves depending on the sky position of the source, the time of the day, and the day of the year. Moreover, the frequency of the emitted signal slowly decreases as the star loses energy, not only in the form of gravitational radiation but possibly also through electromagnetic radiation, an effect known as spin-down.
Our publication describes a search for continuous gravitational waves emitted by neutron stars which are not observed through electromagnetic radiation and which are spinning at frequencies between 10 Hz and 1000 Hz. This corresponds to gravitational-wave frequencies of 20 Hz to 2000 Hz. The low-frequency results were previously published. For this new search, we have again used data from the first observing run (O1) of the Advanced LIGO detectors. O1 achieved a dramatic improvement in sensitivity to gravitational waves with respect to previous science runs of the initial LIGO and Virgo detectors (see, e.g. here or here). Although roughly 2500 neutron stars are observable through emission of electromagnetic radiation — mainly in the radio, gamma-ray and X-ray bands — a much larger number of neutron stars, of the order of 100 million or more, is expected to exist in the Milky Way. Such stars are not visible through their electromagnetic emission because it is either too faint or not pointed toward Earth. A significant number of them, however, could emit gravitational waves detectable by the advanced gravitational-wave detectors.
The search for such signals is complicated by the lack of any prior information on the source: its sky position, gravitational-wave frequency and spin-down are unknown. This means that a large number of possible combinations of these parameters must be explored in order not to miss any potential signal. The optimal data analysis strategy to extract the faint continuous-wave signals from the detector noise is based on “matched filtering” (also used for the search of the transient signals emitted by coalescing binary systems). However, matched filtering becomes computationally prohibitive when data stretches of the order of months or years are used and many parameter combinations are searched over. Hence, to search for these signals with the available computing power, “hierarchical” approaches have been developed in which the entire data set is broken into shorter segments, covering about half an hour to about two hours, each of which is independently analyzed. Afterwards, the information from the different segments is combined. More specifically, for the search described here three different hierarchical methods have been used: PowerFlux, SkyHough, and TimeDomainFstat. These methods differ in several important aspects — like the nature of how the segments are combined, the kind of cleaning procedures used to remove instrumental artifacts from the data, the way to follow-up interesting signal candidates, and the procedure used to compute upper limits on signal strain.
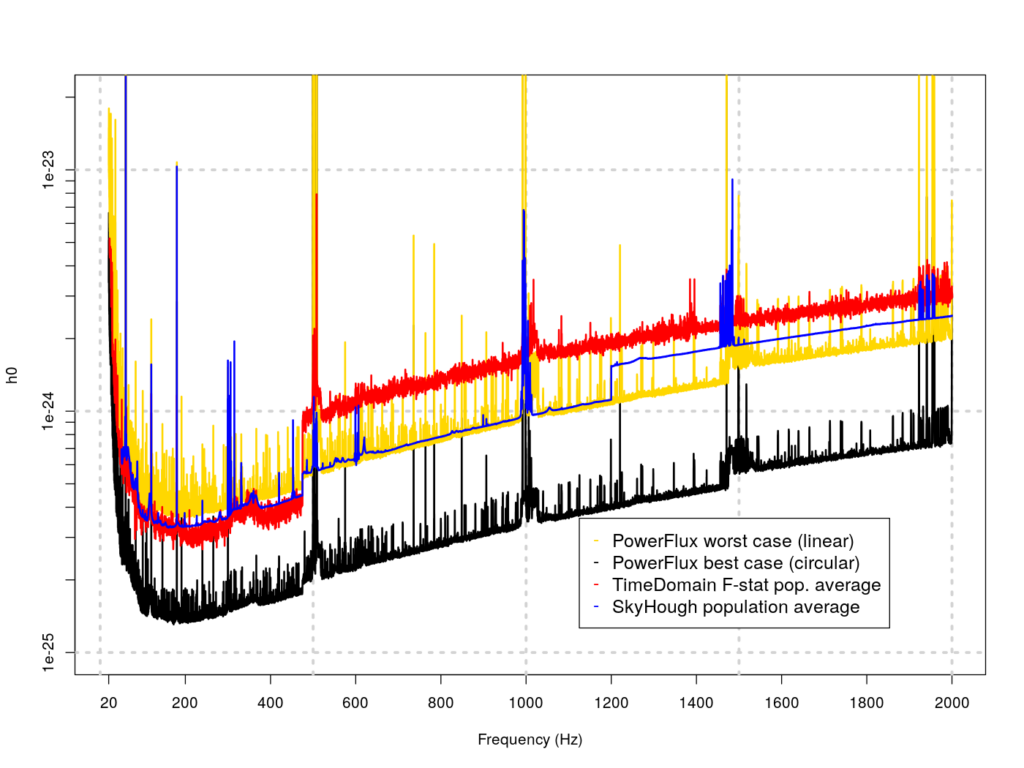
Figure 1: Upper limits on signal amplitude as a function of the frequency. The different symbols/colors correspond to the upper limits computed by the different pipelines.
We have applied all these methods to a search of continuous gravitational-wave signals in O1 data from the entire sky and over a large range of spin-downs between -1.0 × 10-8 Hz/s to 1.0 × 10-9 Hz/s. The results of our analyses do not show any evidence for the presence of a continuous-wave signal in the data set. However, we can still establish upper limits on the signal amplitude, i.e., limits on how strong a signal could be and not be detected (Figure 1). For example, at a frequency of 200 Hz we can exclude the presence of signals with gravitational-wave strain amplitude of ~ 3 × 10-25 or higher with a 95% confidence level. The second figure shows the so-called “astrophysical reach” of the search, which is the relation between the frequency, spin-down and distance for a potentially detectable source. For instance, a source with an ellipticity larger than roughly 2 × 10-7 and emitting a signal at 1500 Hz or larger would be detectable in our analyses if it was closer than 1000 parsecs.
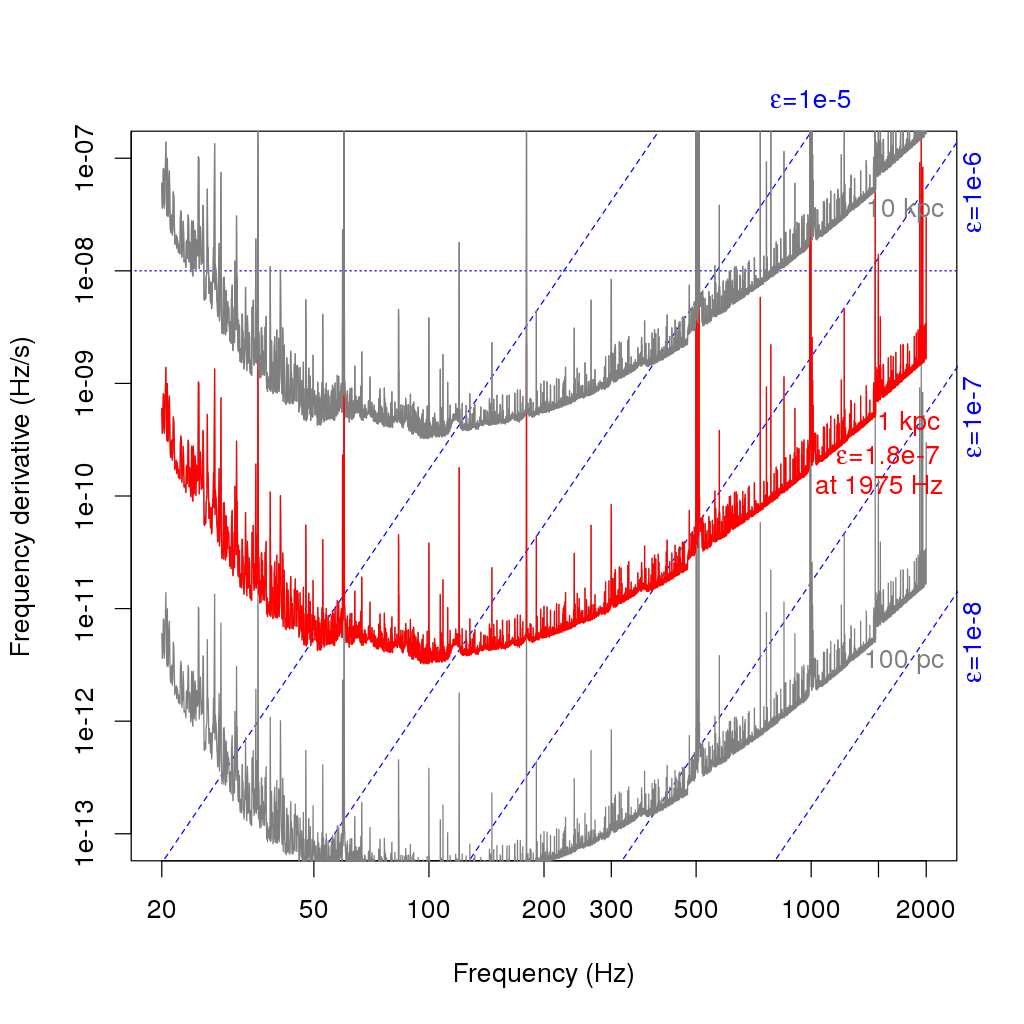
Figure 2: The astrophysical reach of the search shown here is a relation between the frequency derivative and the frequency of a gravitational-wave signal emitted by a detectable source placed at various distances. The lower, medium, and upper curves correspond respectively to a distance of 100 parsecs, 1000 parsecs, and 10,000 parsecs. The dashed lines represent lines of constant ellipticity. The thin horizontal dotted line indicates the maximum spin-down value searched in the analyses. A source with an ellipticity larger than roughly 10-6 and emitting a signal at 200 Hz would be detectable in our analyses at distances below about 400 parsecs if its gravitational-wave-induced spin-down was larger, in modulus, than about 5 × 10-13 Hz/s.
As the Advanced LIGO and Advanced Virgo detectors increase in sensitivity, and other detectors are added to the ground-based network, the probability of detecting continuous gravitational-wave signals emitted by rapidly rotating neutron stars will significantly increase. The detection of these signals will open a new frontier in the study of these fascinating compact objects.
Read more:
- Freely readable preprint of the paper describing the details of the full analysis and results: Full Band All-sky Search for Periodic Gravitational Waves in the O1 LIGO Data by Abbott, et al.