In 2015 the Advanced Laser Interferometer Gravitational Wave Observatory (LIGO) detected gravitational waves from merging black holes (GW150914 and GW151226) during its first observing run. Although these signals originated from distances of more than a billion light years, they were loud enough to be successfully detected despite being within LIGO’s frequency band for less than a second immediately before the black holes’ catastrophic collision. We describe such short-lived signals as ‘transient’.
We are also searching for quieter but long-lasting gravitational waves from another kind of source that can be much closer to us: a rapidly spinning neutron star featuring a small distortion, which is not symmetric as it rotates, is a possible source of continuous gravitational waves. Although sources like this are expected to be very weak, the resulting gravitational-wave signals that they produce are persistent, so that prolonged measurements can try to extract these periodic signals from the randomly varying noise background.
Many neutron stars have been found in binary orbital systems. A Low-mass X-ray binary (LMXB) is a system consisting of a neutron star or a black hole in a binary orbit with a normal star with lower mass (usually a star like our Sun). In these systems the low-mass companion star is drawn into the strong gravitational field of the massive, compact star, feeding it with its shredded mass layers. This process, known as accretion, produces high-energy electromagnetic radiation like X-rays which can be well observed. Neutron stars in LMXB systems are interesting sources of continuous gravitational waves because accretion provides a natural method of deforming the neutron star away from being symmetric as it rotates, powering the gravitational-wave emission. Our theoretical understanding of the process suggests there is a balance between the accretion spinning up the neutron star and the gravitational-wave emission slowing it down. In this sense, a LMXB that is brighter in X-rays may produce stronger gravitational waves.
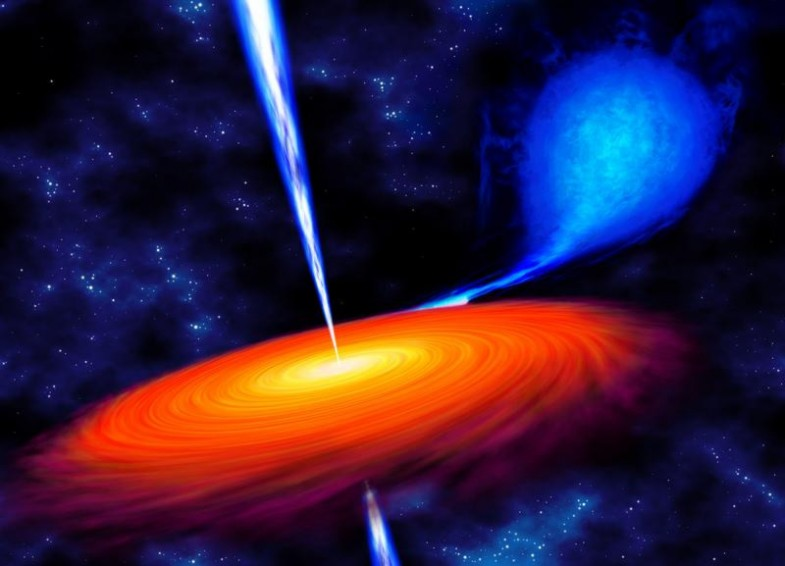
An artist’s impression of the Scorpius X-1 LMXB system. (Courtesy of Ralf Schoofs)
Scorpius X-1, only 9000 light years away, is the brightest LMXB in our galaxy, and hence is potentially one of the strongest continuous gravitational-wave emitters detectable by ground-based detectors like LIGO and Virgo. It is well observed across the electromagnetic spectrum, providing accurate information like its sky position and orbital period. However, there are several challenges in searching for gravitational waves from Scorpius X-1. First, the spin frequency (rotation rate) of the neutron star is unknown, so we cannot predict the gravitational-wave signal frequency. We have to search a broad range of frequencies, which means that the search requires much more computing power. Second, we expect that, in any case, the spin frequency of the neutron star is “wandering”: i.e. constantly changing because of the fluctuations in the amount of accretion from its low-mass companion. This makes it harder to track any signal in the prolonged measurements. Third, because of the orbital motion of the binary system, the signal power is distributed into sidebands – frequencies higher or lower than the gravitational-wave signal frequency.
We have implemented a new method based on a hidden Markov model (HMM), which has already been widely used in engineering applications like radar analysis and mobile telephony, to search efficiently for continuous gravitational waves from the neutron star in Scorpius X-1. We first collect the signal power distributed in the sidebands because of the binary orbital motion, and then track the possible wandering signal using the HMM. The search is conducted using the data collected by the first Advanced LIGO observing run, covering a band of frequencies from 60 Hz to 650 Hz.
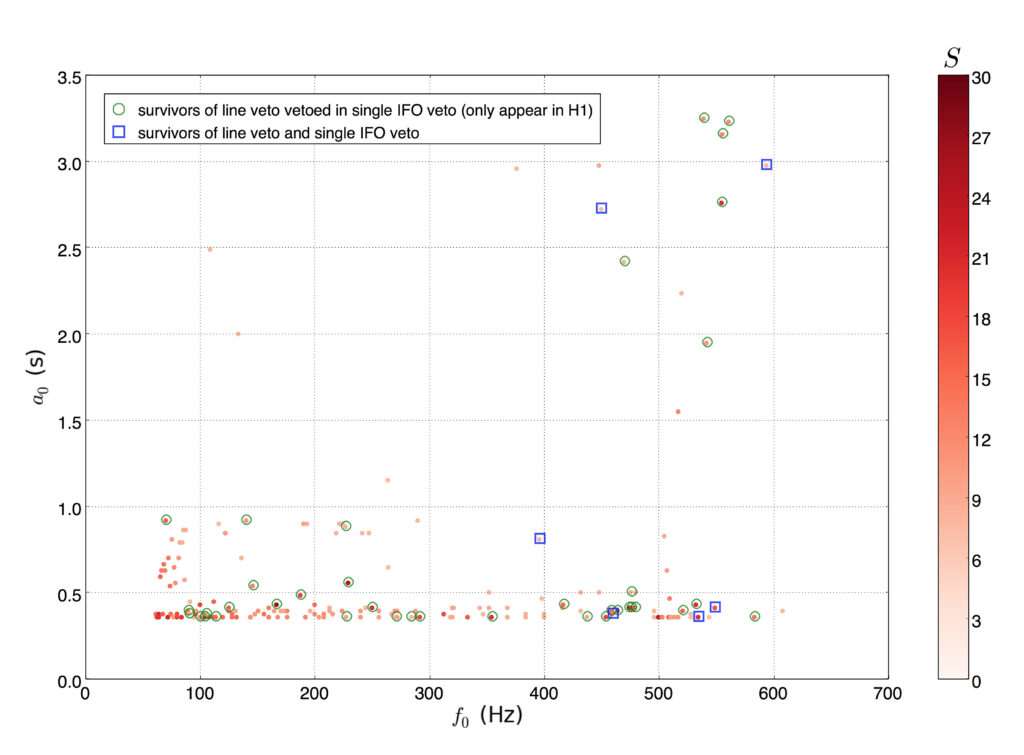
This figure shows the candidate signals identified by the search. Each red dot represents one candidate, whose “detection score” (which provides a measure of the strength of the candidate signal) is above the chosen threshold (detection score > 7.34), in a 1-Hz frequency band indicated by the horizontal axis. The vertical axis of the figure indicates the estimated projected semi-major axis of the binary orbit. The colour of the dots represents the detection score (see colour bar at right). Due to instrumental noise contamination, red dots without green circles or blue squares were rejected in the first follow-up stage of the analysis and the ones marked by green circles were rejected in the second stage. The ones marked by blue squares required further investigation, and were rejected in the third and fourth stages.
Unfortunately, we are not able to claim any detection from this search. However, we are able to place very sensitive upper limits on the gravitational-wave strain from the neutron star in Scorpius X-1 in the frequency band analysed. The search is both sensitive and computationally efficient. We expect to achieve better results when Advanced LIGO further improves its sensitivity and when data from future longer observing runs become available.
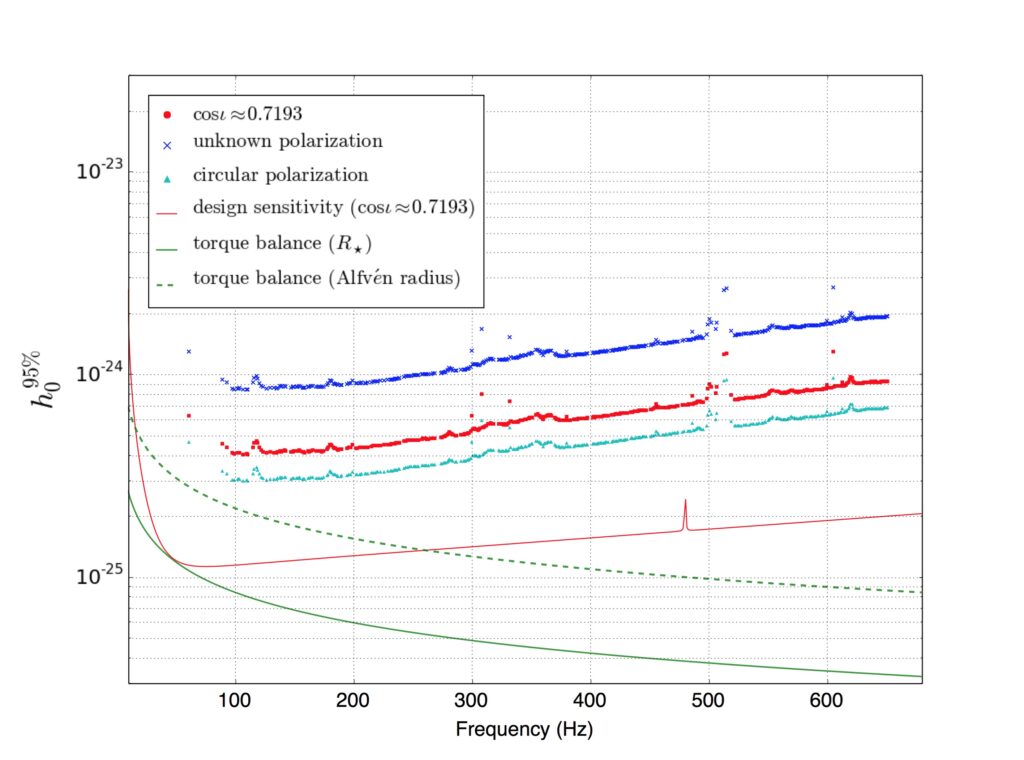
This plot shows the resulting upper limits on the strength of a candidate signal as a function of frequency. The vertical axis represents the gravitational wave strain amplitude. The three types of markers show the results for different assumptions. Blue crosses: we assume that we don’t know the orientation of the neutron star in Scorpius X-1. Red dots: we take the orientation estimated indirectly from electromagnetic (radio) observations. Cyan triangles: we adopt for the neutron star the orientation to which the search is most sensitive. The green solid and dashed curves indicate the theoretical strain amplitudes calculated by assuming a balance between the accretion process and the gravitational-wave emission in two scenarios. The red curve indicates the improved search sensitivity we expect to reach when Advanced LIGO achieves its design sensitivity.
Read more:
- Freely readable preprint of the paper describing the details of the full analysis and results: Search for gravitational waves from Scorpius X-1 in the first Advanced LIGO observing run with a hidden Markov model
- Freely readable preprint of the paper describing the method in more detail: Hidden Markov model tracking of continuous gravitational waves from a neutron star with wandering spin