A previous paper published by the LIGO and Virgo collaborations described a search for continuous gravitational waves from a neutron star called Scorpius X-1. This paper improves on that work in two ways: firstly, by analyzing data from the Laser Interferometer Gravitational-wave Observatory’s (LIGO’s) second observing run, O2 (which ran for a longer period and was slightly more sensitive), and secondly, by using a refined search filter (the J-statistic), capable of more precisely picking out signals from sources like Scorpius X-1 from noisy detector data.
The signals detected so far by LIGO and Virgo gravitational wave detectors have been from mergers of compact objects such as black holes and neutron stars, which are cataclysmic events (usually in distant galaxies) leading to short but very energetic bursts of gravitational waves. Much more elusive have been so-called continuous gravitational waves: gravitational waves that are present all the time, but are much weaker and so can be detected only by a patient search that combines data over a long time span.
This search is specifically aimed at gravitational waves from a neutron star called Scorpius X-1. Neutron stars are extremely dense, rapidly-rotating objects: they pack the mass of the Sun into a sphere with a radius of just ten kilometers (six miles), and which can spin hundreds of times per second. Scorpius X-1 is located 9000 light years from Earth, meaning it’s far away outside our solar system, although still within our Milky Way galaxy. The combination of extreme density and rapid rotation makes systems containing neutron stars promising candidates as continuous gravitational wave sources.
Scorpius X-1 is called a low mass X-ray binary (LMXB), meaning it is a neutron star in a binary orbit with a low-mass normal star (in this case, around 0.4 solar masses), called the companion star. Gas from the companion star is sucked in to the neutron star in a process called accretion, and this accretion process emits X-rays, which can be observed by telescopes from Earth. The brighter the X-rays, the more matter is being accreted on to the neutron star.
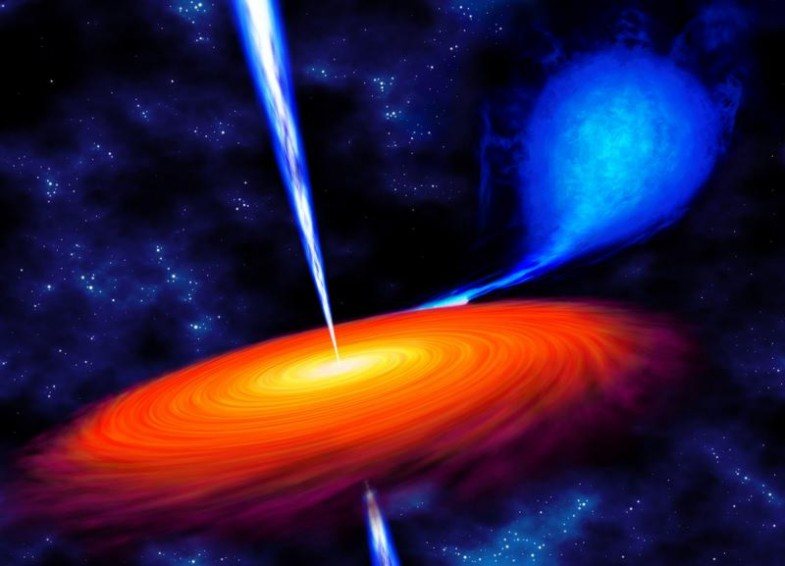
An artist’s impression of the Scorpius X-1 LMXB system. (Courtesy of Ralf Schoofs)
The accretion process is a key piece of the gravitational wave puzzle, because a perfectly smooth neutron star wouldn’t emit gravitational waves: something needs to deform the neutron star, for example, by adding a bump. Accretion is one possible way of making such a deformation, and more accretion suggests more deformation. Scorpius X-1 is the LMXB with the brightest X-rays (in fact, it’s the brightest X-ray source in the sky, other than the Sun), making it an obvious choice of target.
The search itself presents a number challenges. One particular challenge is that, despite many previous observations by X-ray satellites, the number of times the neutron star rotates every second—its spin frequency—has so far proven impossible to measure. The gravitational waves are expected to be at twice the star’s frequency, so this is a crucial input to the search. In this paper, we search for a signal frequency between 60 and 650 Hz (corresponding to spin frequencies between 30 and 325 rotations per second), based on typical spin frequencies of neutron stars known as millisecond pulsars.
Further compounding this problem is that the spin frequency is believed to change over time in an unpredictable way, based on how other LMXBs (where spin frequency can be measured) behave. This phenomenon is called spin wandering
. These frequency changes are very small (about one part in a million) but are still enough to throw off sensitive search methods. We use a technique called a hidden Markov model, which allows us to split the search up into short ten-day segments, individually process each one, and then recombine the short segments in a way that is still sensitive, even if the frequency changes slightly.
A second challenge comes from the binary orbit: because the neutron star is orbiting its companion star, rather than sitting still in the sky, the signal is Doppler shifted. These Doppler shifts make the signal appear to be slightly higher or lower in frequency, depending on where the LMXB is in its orbit. We use a new method, called the J-statistic, which accounts for these frequency shifts. The J-statistic uses measurements of the binary orbit (made with X-ray telescopes) to undo the effect of this Doppler shift on the continuous gravitational wave signal, although key measurements (such as how the orbit of the binary is oriented, relative to the Earth) remain imprecise, which reduces the sensitivity of a search like this one.
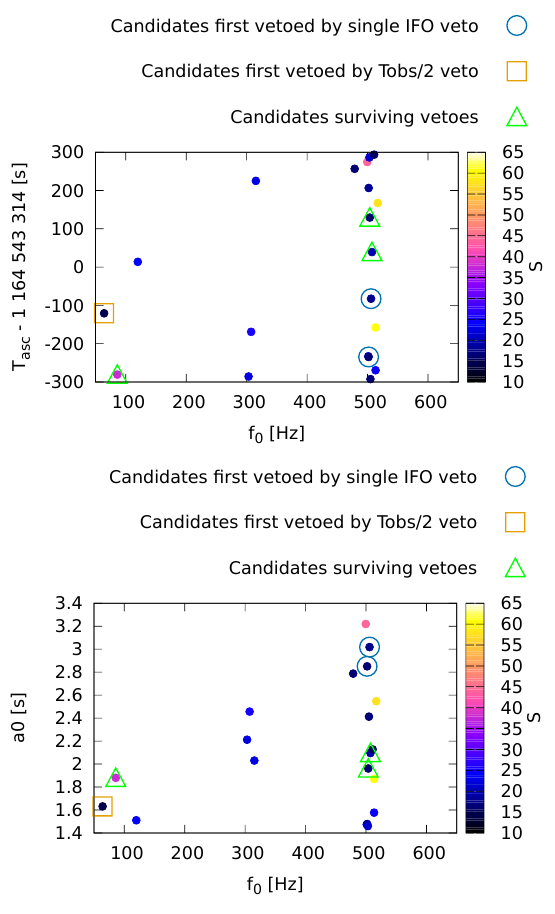
This figure shows possible candidates signals from the search. They are shown based on the signal frequency (horizontal axis) and two different parameters that describe the binary orbit, which we do not know perfectly and so must be searched over (vertical axes on the top and bottom panels). These two parameters are the projected semi-major axis (measuring how wide the orbit appears from Earth) and time of ascension (measuring how far through its orbit the binary is). The color of each marker shows how strong (S) the candidate signal was. Markers not in circles, triangles or squares indicate candidate signals that were rejected because they are found in data that are known to be noisy; markers circled in blue are rejected because they show up in only one detector of the two LIGO detectors, those in orange squares could be found in only the first or second half of the dataset, and those in green triangles survived our pre-established signal tests. The remaining three candidates are judged unlikely to be associated with a signal because they show evidence of being associated with known detector noise features.
No continuous gravitational wave signals were found by the search. Although some signal candidates were found (see the figures), close examination of each one showed that the most likely explanation for each were fluctuations in the background noise inherent in the LIGO detectors, despite their exquisite sensitivity to gravitational waves. Nevertheless, we were able to place upper limits on signals that might be found in the future: that is, the fact this search didn’t find a signal tells us that it must be weaker than the weakest signal we would have been able to detect.
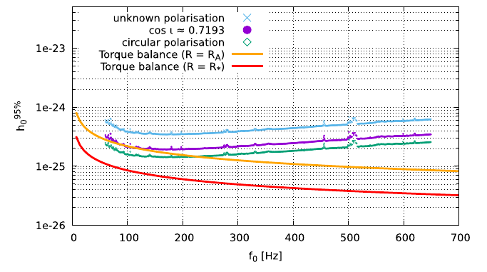
This figure shows the upper limits that have been established by this search on the strength of the gravitational wave signal (vertical axis) coming from Scorpius X-1, depending on the frequency of the signal (horizontal axis). The three markers show the upper limits for three different assumptions about the orientation of the neutron star: at the top, without assuming any knowledge about orientation (light blue); in the middle, an orientation inferred from other observations (purple); and at the bottom, the most optimistic assumption (green). The solid lines show a theoretical calculation of how strong the signal might be, by assuming that all of the energy from accretion is converted into gravitational waves.
Not detecting anything is not too surprising for this search: theoretical calculations suggest that, unless we are extremely lucky, it’s unlikely that the detectors are sensitive enough to detect continuous gravitational waves from Scorpius X-1. Those calculations suggest that the detector will need to be a factor of about 2-5 more sensitive to make a detection.
The search for Scorpius X-1 will continue by analyzing data from Observing Run 3, as the detectors get more sensitive (by reducing detector noise), collect data for a longer time, and we make further improvements to our search algorithms. With these improvements there are good prospects for detecting continuous gravitational waves from Scorpius X-1 and learning more about this fascinating source.
Further reading
- Visit our websites: ligo.org, www.virgo-gw.eu
- Free preprint of the paper discussing the results: Search for gravitational waves from Scorpius X-1 in the second Advanced LIGO observing run with an improved hidden Markov model
- Free preprint of the paper describing the search method in detail: Hidden Markov model tracking of continuous gravitational waves from a binary neutron star with wandering spin. II. Binary orbital phase tracking
- Free preprint of the paper describing the previous Viterbi-based search, on O1 data: Search for gravitational waves from Scorpius X-1 in the first Advanced LIGO observing run with a hidden Markov model